Electricity and Magnetism: The Forces That Power Modern Life
Electricity and magnetism are foundational pillars in the study of physics, governing a vast range of natural and technological phenomena. This domain explains how charged particles interact, how electric and magnetic fields are generated, and how energy propagates in the form of waves. It provides critical insight into the workings of electrical circuits, and how circuit elements are modeled and analyzed.
At the theoretical core lies electrodynamics, which unifies electric and magnetic fields into a coherent framework. Phenomena like electromagnetic induction reveal how changing magnetic fields generate electric currents, a principle exploited in power generation and transformers. The generation and propagation of electromagnetic waves form the basis of wireless communication and optical technologies.
The study of electrostatics lays the groundwork for understanding static charges, while magnetic fields explore the spatial influence of magnets and currents. Broader interdisciplinary applications emerge in magnetohydrodynamics and magnetostatics, where magnetic effects combine with fluid dynamics and equilibrium systems.
Exotic states of matter, such as those studied in plasma physics, rely heavily on electrical and magnetic interactions at high energy levels. Quantum extensions like quantum electrodynamics (QED) delve into particle-field interactions at the smallest scales. Technologies like superconductivity showcase the surprising behaviors of electrons in specially prepared materials.
Electricity and magnetism also intersect powerfully with light and optics. In atmospheric and environmental optics, electromagnetic theory explains the scattering and absorption of sunlight. Innovations in bio-optics and fiber optics have revolutionized medical imaging and high-speed communication.
Core optical theories such as geometrical optics, laser optics, and nonlinear optics build on electromagnetic principles to design lenses, lasers, and signal processors. Fields like photonics and quantum optics probe deeper into the dual particle-wave nature of light.
Wave-based perspectives, developed in wave optics, offer complementary insights into diffraction and interference. The integration of electricity and magnetism with quantum mechanics and relativity leads to the exciting advances now explored in modern physics, where traditional concepts are refined and extended by experimental discovery.
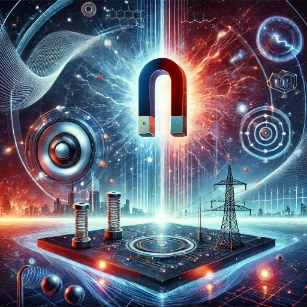
Table of Contents
Key Concepts in Electricity and Magnetism
Electromagnetic Waves
- Description: Electromagnetic waves are oscillating electric and magnetic fields that propagate through space at the speed of light.
- Key Topics:
- Wave properties: Wavelength, frequency, and speed.
- Spectrum: Radio waves, microwaves, infrared, visible light, ultraviolet, X-rays, and gamma rays.
- Applications: Wireless communication, medical imaging (X-rays), and satellite technology.
- Significance: These waves are essential for modern technologies like Wi-Fi, radio, and cellular networks.
Electric Circuits
- Description: Electric circuits involve the flow of electric current through conductive pathways, controlled by components like resistors, capacitors, and inductors.
- Key Topics:
- Ohm’s Law: The relationship between current, voltage, and resistance (
- Kirchhoff’s Laws: Conservation of charge and energy in circuits.
- AC/DC circuits: Alternating current (AC) and direct current (DC) systems.
- Ohm’s Law: The relationship between current, voltage, and resistance (
- Applications:
- Design and optimization of electronic devices.
- Power distribution in homes and industries.
- Significance: Electric circuits are the building blocks of all electronic devices, from simple lightbulbs to complex microprocessors.
Magnetic Fields
- Description: Magnetic fields are produced by moving electric charges and are fundamental to understanding electromagnetism.
- Key Topics:
- Magnetic force: The interaction between magnetic fields and electric currents or magnetic materials.
- Electromagnetic induction: Faraday’s Law, describing how a changing magnetic field induces an electric current.
- Magnetic materials: Ferromagnetism, diamagnetism, and paramagnetism.
- Applications:
- Electric motors and generators.
- Magnetic storage devices like hard drives.
- Significance: Magnetic fields are integral to energy conversion technologies and data storage systems.
Applications of Electricity and Magnetism
Power Generation and Distribution
- Description: Electricity generation involves converting mechanical energy into electrical energy, typically using magnetic fields.
- Key Technologies:
- Generators: Use electromagnetic induction to produce electricity in power plants.
- Transformers: Step up or step down voltage levels for efficient power transmission.
- Grid systems: Distribute electricity from power plants to consumers.
- Significance: Reliable power systems enable industrial production, home utilities, and economic development.
Electronics Development
- Description: The principles of electricity and magnetism form the foundation for designing and manufacturing electronic devices.
- Key Technologies:
- Semiconductors: Used in transistors and integrated circuits.
- Wireless communication: Enabled by electromagnetic waves (e.g., Wi-Fi, Bluetooth, cellular networks).
- Display technologies: LCD, OLED, and touchscreens.
- Applications:
- Smartphones, computers, and smart home devices.
- Advanced medical equipment like MRI scanners.
- Significance: Electronics have revolutionized communication, healthcare, and entertainment.
Transportation Systems
- Description: Electric and magnetic technologies power modern transportation systems.
- Key Technologies:
- Electric vehicles (EVs): Use electric motors powered by rechargeable batteries.
- Maglev trains: Employ magnetic levitation to achieve high-speed, frictionless travel.
- Significance: These systems are transforming the transportation industry by offering sustainable and efficient solutions.
Scientific and Medical Applications
- Description: Electricity and magnetism are pivotal in scientific research and healthcare technologies.
- Key Technologies:
- Particle accelerators: Study subatomic particles using electromagnetic fields.
- MRI (Magnetic Resonance Imaging): Non-invasive imaging for medical diagnosis.
- Lasers: Used in surgery, research, and communication.
- Significance: These applications drive innovation in medicine, physics, and material science.
Modern Relevance of Electricity and magnetism
Electricity and magnetism are indispensable in today’s interconnected world. They underpin innovations in renewable energy, smart grids, and advanced electronics. Research into superconductors, quantum computing, and electromagnetic shielding continues to expand the boundaries of knowledge and application.
Sub-Areas of Studies in Electricity and Magnetism
Electricity and magnetism is a vast field of physics, encompassing multiple sub-areas that focus on different aspects of electric and magnetic phenomena. These subfields explore the behavior, interaction, and applications of electric charges, currents, and magnetic fields. Below are the key sub-areas of study:Electrostatics
- Focus: The study of stationary electric charges and the electric fields they produce.
- Key Topics:
- Coulomb’s Law: Interaction between charged particles.
- Electric field and electric potential.
- Capacitance and electrostatic energy storage.
- Applications:
- Design of capacitors and electrostatic precipitators.
- Analysis of charges in insulating materials and lightning phenomena.
Electrodynamics
- Focus: The study of moving electric charges and the resulting electric currents and magnetic fields.
- Key Topics:
- Ohm’s Law and electric circuits.
- Kirchhoff’s Circuit Laws for complex electrical networks.
- Electromotive force (EMF) and electrical resistance.
- Applications:
- Power transmission systems and electric motors.
- Circuit design for electronics and communication systems.
Magnetostatics
- Focus: The study of magnetic fields produced by steady (unchanging) electric currents.
- Key Topics:
- Biot-Savart Law and Ampère’s Law.
- Magnetic field lines and magnetic flux.
- Magnetic materials and their properties (ferromagnetism, diamagnetism, paramagnetism).
- Applications:
- Magnetic levitation (Maglev) systems.
- Design of electromagnets and transformers.
Electromagnetic Induction
- Focus: The study of how changing magnetic fields induce electric currents and vice versa.
- Key Topics:
- Faraday’s Law of Electromagnetic Induction.
- Lenz’s Law: Direction of induced currents.
- Inductance and self-inductance.
- Applications:
- Power generation in alternators and generators.
- Wireless charging technologies and induction heating.
Electromagnetic Waves
- Focus: The study of oscillating electric and magnetic fields that propagate as waves.
- Key Topics:
- Maxwell’s Equations and the unification of electricity and magnetism.
- Wave properties: Frequency, wavelength, and speed.
- Electromagnetic spectrum: Radio waves, microwaves, visible light, X-rays, and gamma rays.
- Applications:
- Wireless communication (radio, Wi-Fi, cellular networks).
- Satellite and radar technologies.
- Medical imaging, such as X-rays and MRIs.
Electrical Circuits
- Focus: The study of closed-loop systems where electric current flows.
- Key Topics:
- Circuit components: Resistors, capacitors, and inductors.
- AC/DC circuits: Alternating and direct current analysis.
- Circuit theory and network theorems (Thevenin’s and Norton’s theorems).
- Applications:
- Designing electronics and appliances.
- Optimizing energy efficiency in power systems.
Magnetohydrodynamics (MHD)
- Focus: The study of the behavior of electrically conducting fluids (e.g., plasmas, liquid metals) in magnetic fields.
- Key Topics:
- Interaction between fluid flow and magnetic fields.
- Magnetic Reynolds number and Alfvén waves.
- Applications:
- Astrophysical studies of stars and planetary magnetospheres.
- Fusion reactors and cooling systems for nuclear reactors.
Plasma Physics
- Focus: The study of ionized gases and their interactions with electric and magnetic fields.
- Key Topics:
- Plasma confinement and stability.
- Wave-particle interactions in plasmas.
- Magnetospheres and space plasmas.
- Applications:
- Controlled nuclear fusion.
- Spacecraft propulsion systems (e.g., ion thrusters).
Superconductivity
- Focus: The study of materials that exhibit zero electrical resistance and expel magnetic fields at low temperatures.
- Key Topics:
- Meissner effect and flux quantization.
- Type I and Type II superconductors.
- High-temperature superconductors.
- Applications:
- Magnetic levitation (Maglev) trains.
- Superconducting magnets in MRI machines and particle accelerators.
Quantum Electrodynamics (QED)
- Focus: The quantum study of electromagnetic interactions at the particle level.
- Key Topics:
- Interaction between photons and charged particles.
- Quantum fields and Feynman diagrams.
- Vacuum polarization and radiative corrections.
- Applications:
- High-precision atomic clocks.
- Quantum computing and cryptography.
Why Study Electricity & Magnetism
Fundamental Forces in Nature
Electricity and magnetism describe two aspects of a fundamental interaction in physics. Students explore electric charges, fields, currents, and magnetic forces. These concepts are key to understanding how the universe operates. They reveal deep connections between matter and energy.
Applications in Technology and Society
Students see electricity and magnetism in action through power generation, communication, and medical devices. Understanding these principles supports innovation in modern technology. This includes motors, transformers, and data transmission systems. It connects theoretical science with everyday life.
Mathematical and Field Theory Approach
Students learn to model electric and magnetic fields using vector calculus. This includes solving Maxwell’s equations and interpreting field lines. These tools support theoretical clarity and practical computation. They provide a gateway to electromagnetics and optics.
Experimentation and Measurement
Hands-on activities include circuit building, field mapping, and electromagnet demonstrations. Students develop skills in observation, instrumentation, and data interpretation. These experiences reinforce conceptual understanding. They also build technical proficiency for scientific and engineering careers.
Foundation for Electrodynamics and Modern Physics
Electricity and magnetism form the basis for electromagnetism and quantum electrodynamics. Students who master this area are prepared for advanced studies in physics and engineering. This supports careers in research, electronics, and telecommunications. It connects classical and modern scientific thought.
Conclusion on Electricity and Magnetism
The study of electricity and magnetism reveals the fundamental forces that shape our technological and natural world. By understanding electric and magnetic fields and their interactions, scientists and engineers have created transformative technologies that power our homes, connect our devices, and improve our quality of life. With ongoing advancements, the principles of electricity and magnetism will continue to play a vital role in shaping the future.
Electricity and Magnetism: Review Questions and Answers
1. What is electricity and how is it characterized in physics?
Answer: Electricity is the study of the behavior and effects of electric charges. It is characterized by concepts such as electric current, voltage, resistance, and the behavior of conductors and insulators.
2. How do electric fields influence charged particles?
Answer: Electric fields exert forces on charged particles, causing them to accelerate. The force experienced by a charge is given by F = qE, where q is the charge and E is the electric field strength.
3. What is Ohm’s law and why is it fundamental to circuit analysis?
Answer: Ohm’s law states that the current flowing through a conductor is directly proportional to the voltage across it and inversely proportional to its resistance (V = IR). This law is essential for analyzing and designing electrical circuits.
4. How does a capacitor store energy in an electric field?
Answer: A capacitor stores energy by accumulating electric charge on its plates, which creates an electric field between them. The stored energy is given by the formula U = ½CV², where C is the capacitance and V is the voltage.
5. What are the differences between conductors and insulators?
Answer: Conductors allow electric charges to move freely due to a high density of free electrons, while insulators resist the flow of electric charge because their electrons are tightly bound to atoms.
6. How do magnetic fields interact with moving charges?
Answer: Magnetic fields exert a force on moving charges perpendicular to both the direction of motion and the magnetic field, as described by the Lorentz force law: F = q(v × B).
7. What is electromagnetic induction and how does it work?
Answer: Electromagnetic induction is the process of generating an electric current by changing the magnetic flux through a circuit. Faraday’s law quantifies this effect, stating that the induced electromotive force (EMF) is proportional to the rate of change of magnetic flux.
8. How are alternating current (AC) and direct current (DC) different?
Answer: In direct current (DC), the electric charge flows in one direction, while in alternating current (AC), the direction of current reverses periodically. This difference affects how energy is transmitted and used in electrical systems.
9. What role do transformers play in electrical circuits?
Answer: Transformers use electromagnetic induction to change the voltage level in AC circuits. They can step up or step down voltage, making it possible to efficiently transmit power over long distances.
10. How is the concept of energy conservation applied in electrical circuits?
Answer: In electrical circuits, energy conservation means that the total energy supplied by sources equals the energy consumed by loads and lost as heat. This principle is used to analyze and design circuits to ensure efficiency and safety.
Electricity and Magnetism: Thought-Provoking Questions and Answers
1. How does the microscopic behavior of electrons in conductors give rise to macroscopic electrical properties?
Answer: The collective motion of electrons, which are influenced by atomic structure and impurities, determines conductivity and resistance. This microscopic behavior is explained by quantum mechanics and statistical physics, linking electron mobility to macroscopic phenomena like current flow.
2. In what ways might the development of superconductors revolutionize electrical power transmission?
Answer: Superconductors, which exhibit zero electrical resistance at low temperatures, could dramatically reduce energy losses during power transmission. Their use could lead to more efficient grids, miniaturized electronics, and advances in magnetic levitation technologies.
3. How can the interplay between electricity and magnetism be harnessed in modern technology?
Answer: The interaction between electric and magnetic fields is fundamental to devices such as motors, generators, and transformers. Innovations leveraging this interplay are driving the development of renewable energy technologies, wireless charging systems, and advanced computing components.
4. What are the environmental and societal impacts of transitioning from fossil fuel-based power generation to renewable electrical energy sources?
Answer: Shifting to renewable energy reduces greenhouse gas emissions, mitigates climate change, and decreases pollution. It also promotes energy independence, creates new job opportunities in green technologies, and fosters sustainable development on a global scale.
5. How does electromagnetic induction enable wireless energy transfer, and what are its limitations?
Answer: Electromagnetic induction allows energy to be transferred through oscillating magnetic fields between coils. Wireless energy transfer is used in applications like charging pads and RFID systems, but its efficiency drops with distance and alignment challenges limit its widespread adoption.
6. What challenges do engineers face when designing circuits that must operate under high-frequency AC conditions?
Answer: At high frequencies, factors such as parasitic capacitance and inductance become significant, leading to signal distortion and losses. Engineers must carefully design circuit layouts, use appropriate materials, and employ advanced modeling techniques to mitigate these effects.
7. How can advanced materials improve the performance and durability of capacitors and batteries?
Answer: Advanced materials with higher dielectric constants, better conductivity, or enhanced chemical stability can increase energy storage capacity and longevity. Innovations in nanomaterials and solid electrolytes are leading to more efficient, compact, and durable energy storage devices.
8. In what ways does the study of electromagnetism contribute to our understanding of the universe at large scales?
Answer: Electromagnetism explains phenomena ranging from the behavior of plasma in stars to the dynamics of galaxies and cosmic magnetic fields. It is integral to astrophysics and cosmology, providing insights into the structure, evolution, and interactions of celestial bodies.
9. How might emerging quantum technologies leverage the principles of electricity and magnetism to create new computing paradigms?
Answer: Quantum technologies use phenomena like superposition and entanglement, which are deeply connected to electromagnetic interactions, to develop quantum bits (qubits) and circuits. These advances promise to revolutionize computing by solving complex problems far more efficiently than classical systems.
10. How does the concept of energy conversion in transformers illustrate the principle of conservation of energy?
Answer: Transformers convert energy from one voltage level to another without loss (ideally) by using electromagnetic induction. This conversion obeys the conservation of energy, where the input electrical energy equals the sum of the output energy and any losses due to inefficiencies.
11. What are the potential benefits and challenges of integrating renewable energy sources into the existing electrical grid?
Answer: Benefits include reduced emissions, energy diversity, and sustainability. Challenges involve managing variable power outputs, ensuring grid stability, and upgrading infrastructure to handle decentralized and intermittent energy production.
12. How can interdisciplinary approaches enhance our understanding of complex electromagnetic phenomena in emerging technologies?
Answer: Combining expertise from physics, materials science, electrical engineering, and computer science enables the development of sophisticated models and experimental techniques. This interdisciplinary approach fosters innovations in fields like wireless communication, medical imaging, and renewable energy systems, leading to breakthroughs that might not be possible within a single discipline.
Electricity and Magnetism: Numerical Problems and Solutions:
1. A circuit has a resistor of 100 Ω connected to a 12 V battery. Calculate the current flowing through the circuit.
Solution:
Using Ohm’s law: I = V/R = 12 V / 100 Ω = 0.12 A.
2. Two resistors, 200 Ω and 300 Ω, are connected in series. Determine the equivalent resistance and the total current from a 24 V battery.
Solution:
Equivalent resistance, R_eq = 200 Ω + 300 Ω = 500 Ω.
Current, I = V/R_eq = 24 V / 500 Ω = 0.048 A.
3. A capacitor of 50 μF is charged to a voltage of 10 V. Calculate the energy stored in the capacitor.
Solution:
Energy, U = ½ C V² = 0.5 × 50×10⁻⁶ F × (10 V)² = 0.5 × 50×10⁻⁶ × 100 = 0.0025 J.
4. A coil with an inductance of 0.2 H is connected to a 5 V DC source. If the coil initially has no current, calculate the time constant τ of the RL circuit.
Solution:
Time constant, τ = L/R.
Assuming resistance R = 10 Ω, τ = 0.2 H / 10 Ω = 0.02 s.
Note: Without an R value provided, a typical assumption (e.g., 10 Ω) is made for illustration.
5. An AC circuit has an RMS voltage of 120 V and a resistance of 60 Ω. Calculate the RMS current.
Solution:
Using Ohm’s law for RMS values: I_RMS = V_RMS / R = 120 V / 60 Ω = 2 A.
6. A transformer has a primary coil with 500 turns and a secondary coil with 100 turns. If the primary voltage is 240 V, what is the secondary voltage (assuming ideal conditions)?
Solution:
Transformer ratio: V_s/V_p = N_s/N_p, so V_s = V_p × (N_s/N_p) = 240 V × (100/500) = 240 V × 0.2 = 48 V.
7. Calculate the magnetic force on a 3 A current-carrying wire of length 0.5 m placed in a magnetic field of 0.4 T, if the wire is perpendicular to the field.
Solution:
Magnetic force, F = I L B = 3 A × 0.5 m × 0.4 T = 0.6 N.
8. An electron moves in a uniform magnetic field of 0.2 T with a velocity of 2×10⁶ m/s perpendicular to the field. Calculate the radius of its circular path (electron mass m = 9.11×10⁻³¹ kg, charge e = 1.6×10⁻¹⁹ C).
Solution:
Radius, r = (m v)/(e B) = (9.11×10⁻³¹ kg × 2×10⁶ m/s) / (1.6×10⁻¹⁹ C × 0.2 T) ≈ (1.822×10⁻²⁴) / (3.2×10⁻²⁰) ≈ 5.69×10⁻⁵ m.
9. A resistor and capacitor are connected in series (RC circuit) with R = 1 kΩ and C = 10 μF. Calculate the time constant τ of the circuit.
Solution:
Time constant, τ = R × C = 1000 Ω × 10×10⁻⁶ F = 0.01 s.
10. In an RLC circuit, the resonant frequency is given by f₀ = 1/(2π√(LC)). If L = 0.05 H and C = 20 μF, determine f₀.
Solution:
f₀ = 1/(2π√(0.05 × 20×10⁻⁶)) = 1/(2π√(1×10⁻⁶)) = 1/(2π×0.001) ≈ 159.15 Hz.
11. A solenoid has 500 turns, a length of 0.5 m, and carries a current of 2 A. Calculate the magnetic field inside the solenoid (μ₀ = 4π×10⁻⁷ T·m/A).
Solution:
Magnetic field, B = μ₀ (N/L) I = 4π×10⁻⁷ T·m/A × (500/0.5 m) × 2 A
= 4π×10⁻⁷ × 1000 × 2 = 8π×10⁻⁴ T ≈ 0.00251 T.
12. A parallel RC circuit has a resistor of 200 Ω and a capacitor of 100 μF. If the voltage across the circuit is 10 V, calculate the initial charging current through the resistor when the capacitor is uncharged.
Solution:
Initially, the capacitor behaves like a short circuit, so the current, I = V/R = 10 V / 200 Ω = 0.05 A.