Introduction to Physical Chemistry
Physical chemistry is the branch of chemistry that blends the principles of physics and chemical systems to explain the fundamental mechanisms behind matter and energy. It provides the mathematical and theoretical framework to analyze chemical phenomena such as thermodynamics, kinetics, quantum chemistry, and molecular interactions. By integrating concepts from science, students gain a holistic view of how atomic and molecular systems behave under various physical conditions.
This field is crucial for understanding the dynamics of life at the molecular level. In biochemistry and biology, for instance, the rates of metabolic reactions, diffusion of ions across membranes, and energy transformations all depend on physical chemistry principles. Topics like energy and metabolism and homeostasis rely heavily on thermodynamic and equilibrium models, while signal propagation in neurons—explored in signal communication—involves electrochemical gradients and transport kinetics.
Beyond living systems, physical chemistry supports the advancement of technologies in industrial chemistry through the optimization of reaction conditions and catalyst design. Understanding chemical kinetics and thermodynamics also strengthens the design of efficient industrial processes and environmentally friendly alternatives. This has deep relevance to environmental chemistry and applications like emissions control and pollutant modeling.
Moreover, physical chemistry underpins topics in analytical chemistry, particularly in the interpretation of spectroscopic data, calorimetry, and electrochemical techniques. These analytical methods are essential in fields such as conservation science, climate science, and environmental science, where precise measurement and prediction of environmental variables are vital.
Theoretical models in physical chemistry also enhance our understanding of geological and atmospheric systems. For example, phase transitions and solubility principles are essential in geology and meteorology. The behavior of gases and fluids within oceanic and atmospheric layers is analyzed using laws from physical chemistry in oceanography. These interconnections illustrate how chemical physics informs our approach to global environmental challenges.
At the interface of physics and chemistry, this field also enhances our understanding of organic and inorganic chemistry, especially in terms of molecular structure, bond energies, and spectroscopy. These principles are also foundational for students exploring structure-function relationships in biomolecules and materials.
Finally, physical chemistry provides critical insights into sustainable technologies and energy transitions. Its principles are central to the advancement of renewable energy studies, including fuel cells, photovoltaics, and battery chemistry. Understanding the physical properties of materials and energy conversion mechanisms empowers innovation toward more sustainable industrial and environmental practices.
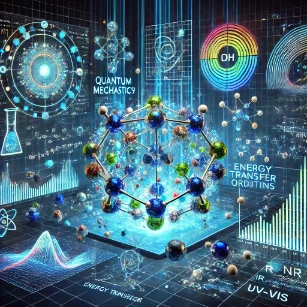
Table of Contents
Key Areas of Physical Chemistry
Thermodynamics
- Examines the relationship between energy, heat, work, and matter in chemical systems.
- Focuses on understanding how energy transformations govern chemical reactions and phase changes.
- Key concepts include:
- Laws of Thermodynamics: Describing energy conservation, entropy, and equilibrium.
- Gibbs Free Energy: Determines whether a reaction will occur spontaneously.
- Phase Diagrams: Illustrate the states of matter under varying temperature and pressure.
Quantum Chemistry
- Applies the principles of quantum mechanics to study the behavior of electrons and atoms.
- Helps predict molecular properties and reaction pathways.
- Key aspects include:
- Electronic Structure: How electrons are distributed in atoms and molecules.
- Molecular Orbitals: Describing bonding and anti-bonding interactions.
- Spectroscopy: Studying molecular energy levels through interactions with electromagnetic radiation.
Chemical Kinetics
- Investigates the rates of chemical reactions and the factors influencing them.
- Explains reaction mechanisms and the role of intermediates.
- Key topics include:
- Rate Laws: Mathematical expressions that describe reaction rates.
- Activation Energy: The energy barrier that must be overcome for a reaction to proceed.
- Catalysis: How catalysts lower activation energy to speed up reactions.
Statistical Mechanics
- Links microscopic molecular behavior to macroscopic thermodynamic properties.
- Provides a statistical basis for understanding entropy, energy distribution, and reaction dynamics.
Molecular Interactions
- Studies how molecules interact through forces like hydrogen bonding, van der Waals forces, and dipole interactions.
- Important for understanding solutions, solubility, and protein-ligand binding.
Applications of Physical Chemistry
Catalysis
- Understanding the mechanisms of catalysts to optimize industrial chemical reactions.
- Examples: Development of catalysts for petroleum refining and polymer production.
Material Science
- Explains the physical properties of materials like semiconductors, polymers, and nanomaterials.
- Applications include designing solar cells, superconductors, and advanced composites.
Pharmaceuticals
- Assists in drug design by modeling molecular interactions and reaction dynamics.
- Example: Using thermodynamic data to predict drug solubility and stability.
Environmental Science
- Studies reactions in the atmosphere, hydrosphere, and biosphere.
- Applications include understanding ozone depletion, greenhouse gases, and water treatment processes.
Energy Conversion and Storage
- Investigates processes like fuel cell reactions, battery efficiency, and photovoltaic systems.
- Example: Optimizing electrochemical reactions in lithium-ion batteries.
Modern Tools and Techniques in Physical Chemistry
Spectroscopic Methods
- Tools like Raman spectroscopy, infrared spectroscopy, and NMR provide insights into molecular structure and dynamics.
Computational Chemistry
- Uses computer simulations to model molecular behavior and predict reaction outcomes.
- Examples: Density Functional Theory (DFT), Molecular Dynamics (MD).
Surface Science
- Explores reactions occurring at interfaces, crucial for catalysis and materials design.
- Techniques include Atomic Force Microscopy (AFM) and Scanning Tunneling Microscopy (STM).
Laser Techniques
- Time-resolved laser spectroscopy tracks reaction dynamics on ultrafast timescales.
- Useful in studying photochemical reactions and energy transfer.
Impact of Physical Chemistry
Physical chemistry plays a vital role in advancing numerous scientific and industrial fields by offering a deep theoretical understanding of chemical processes and practical tools for their application. It provides the foundation for designing and optimizing chemical reactions, enabling researchers to develop new materials, improve energy systems, and innovate in sectors like pharmaceuticals, nanotechnology, and environmental science. The principles of thermodynamics, kinetics, and quantum mechanics allow scientists to manipulate molecular behavior, leading to the discovery of more efficient catalysts, improved drug formulations, and novel polymers with tailored properties. By applying these fundamental concepts, physical chemists contribute to technological progress and the development of high-performance materials used in electronics, aerospace, and medical devices.
One of the most impactful applications of physical chemistry is in energy research and sustainable solutions. The study of reaction kinetics and electrochemistry has led to significant advancements in battery technology, fuel cells, and hydrogen storage, which are crucial for the transition to clean energy sources. Innovations such as lithium-ion batteries, solid-state electrolytes, and solar cells rely on precise control of molecular interactions to maximize energy efficiency and storage capacity. Additionally, physical chemistry plays a key role in developing greener chemical processes by optimizing reaction conditions, minimizing waste, and enhancing selectivity in industrial synthesis. These innovations support sustainability efforts by reducing carbon emissions, improving energy conservation, and creating environmentally friendly materials.
Beyond energy and materials science, physical chemistry is instrumental in understanding and solving complex chemical problems across multiple disciplines. It aids in the development of precise analytical techniques such as spectroscopy, chromatography, and computational modeling, which are essential for studying molecular interactions in pharmaceuticals, biotechnology, and forensic science. In medicine, physical chemistry contributes to drug discovery by modeling protein-ligand interactions and predicting biochemical reaction pathways. In environmental science, it helps analyze pollutants, assess atmospheric chemistry, and improve water purification methods. Whether applied to industrial manufacturing, medical research, or climate science, physical chemistry remains an indispensable field that drives innovation and expands our understanding of the molecular world.
Why Study Physical Chemistry
Linking Chemistry with Physics
Physical chemistry explains chemical phenomena through physical principles like thermodynamics and quantum mechanics. Students study how energy, structure, and motion affect chemical systems. This interdisciplinary field bridges theory with practical understanding. It deepens comprehension of matter and energy interactions.
Thermodynamics and Equilibrium
Students explore the laws of thermodynamics, entropy, and chemical equilibrium. These concepts are vital for understanding reaction spontaneity and system behavior. They apply to energy efficiency, battery design, and chemical engineering. This knowledge supports both academic study and technological innovation.
Kinetics and Reaction Rates
Chemical kinetics investigates how fast reactions occur and what factors influence them. Students learn to measure rate laws and determine mechanisms. This is essential for designing reactors and optimizing industrial processes. It also supports understanding of biological systems and catalysis.
Quantum Chemistry and Molecular Structure
Quantum chemistry uses mathematical models to describe atomic and molecular behavior. Students study orbitals, bonding, and spectroscopy. This helps explain phenomena not covered by classical chemistry. It provides a rigorous framework for advanced scientific work.
Preparation for Research and Graduate Study
Physical chemistry builds strong analytical, mathematical, and conceptual skills. These are essential for careers in research, energy science, and theoretical modeling. Students gain tools for problem-solving and scientific computing. It lays a solid foundation for interdisciplinary study and innovation.
Physical Chemistry: Review Questions and Answers:
What is physical chemistry and how does it differ from other branches of chemistry?
Answer: Physical chemistry is the branch of chemistry that studies the physical properties and underlying principles of chemical systems. It differs from other branches by emphasizing the quantitative relationships between energy, structure, and dynamics, employing mathematical models and experimental techniques to explain chemical behavior.How does thermodynamics explain energy transformations in chemical reactions?
Answer: Thermodynamics focuses on energy changes and the direction of chemical reactions. It uses concepts like enthalpy, entropy, and free energy to predict whether a reaction will occur spontaneously. By analyzing these parameters, chemists can determine the feasibility of reactions and understand how energy is absorbed or released during chemical processes.What is the significance of quantum mechanics in understanding atomic and molecular behavior?
Answer: Quantum mechanics provides a framework for understanding the behavior of particles at the atomic and subatomic levels. It explains electron configurations, orbital shapes, and the distribution of energy within atoms and molecules. This understanding is crucial for predicting chemical bonding, reaction mechanisms, and the spectroscopic properties of substances.How do reaction kinetics help in understanding the rate and mechanism of chemical reactions?
Answer: Reaction kinetics studies the speed at which chemical reactions occur and the steps involved in the process. It identifies factors that affect reaction rates—such as concentration, temperature, and catalysts—and helps elucidate the reaction mechanism by revealing the sequence of elementary steps, intermediates, and transition states involved in the overall reaction.What role does spectroscopy play in analyzing chemical substances?
Answer: Spectroscopy involves the interaction of electromagnetic radiation with matter, allowing scientists to probe the structure and dynamics of molecules. Techniques such as infrared (IR), ultraviolet-visible (UV-Vis), nuclear magnetic resonance (NMR), and mass spectrometry provide detailed information about molecular vibrations, electronic transitions, and chemical environments, making spectroscopy an indispensable tool for chemical analysis.How do physical chemists use mathematical models to predict chemical behavior?
Answer: Mathematical models in physical chemistry, including statistical mechanics and quantum chemical calculations, help predict molecular properties, reaction rates, and equilibrium states. These models allow chemists to simulate complex systems, quantify energy distributions, and understand the fundamental forces driving chemical processes, thereby guiding experimental design and industrial applications.What is the importance of studying phase transitions in physical chemistry?
Answer: Studying phase transitions—such as melting, boiling, and sublimation—provides insight into the changes in physical properties that occur when substances change state. Understanding these transitions helps explain how intermolecular forces operate under varying conditions and informs the design of materials with specific thermal, mechanical, and optical properties.How do intermolecular forces influence the physical properties of substances?
Answer: Intermolecular forces, including hydrogen bonding, van der Waals forces, and dipole-dipole interactions, determine the physical properties of substances such as boiling point, melting point, viscosity, and solubility. These forces affect how molecules aggregate, interact, and respond to external conditions, thereby shaping the macroscopic behavior of materials.How can the study of physical chemistry contribute to advancements in material science?
Answer: Physical chemistry provides a deep understanding of the interactions and energy exchanges at the molecular level, which is essential for designing new materials. By elucidating the principles behind phase transitions, molecular dynamics, and electronic structure, physical chemists can engineer materials with tailored properties for use in electronics, nanotechnology, and energy storage.What are some experimental techniques used in physical chemistry to measure thermodynamic properties?
Answer: Experimental techniques such as calorimetry, spectroscopy, and pressure-volume-temperature (PVT) measurements are used to determine thermodynamic properties. Calorimetry measures heat changes during reactions, while spectroscopic methods provide insights into molecular energy states. PVT experiments help establish equations of state for gases and liquids, all of which are vital for understanding energy transformations.
Physical Chemistry: Thought-Provoking Questions and Answers
How does the interplay between thermodynamics and kinetics determine the feasibility and rate of a chemical reaction?
Answer: Thermodynamics and kinetics, though distinct, jointly influence a chemical reaction. Thermodynamics tells us if a reaction is energetically favorable by evaluating free energy changes, while kinetics reveals how fast the reaction will proceed by analyzing the energy barrier (activation energy). A reaction may be thermodynamically favorable yet proceed slowly if the activation energy is high. Conversely, a kinetically fast reaction might be non-spontaneous unless driven by specific conditions, highlighting the importance of both aspects in designing efficient chemical processes.In what ways can quantum mechanical principles be applied to predict chemical reactivity and bonding?
Answer: Quantum mechanics allows chemists to calculate electron distributions, energy levels, and orbital interactions that underpin chemical bonds. By using models such as molecular orbital theory and density functional theory, one can predict bond strengths, reaction sites, and molecular geometries. These predictions help in understanding reactivity patterns, designing novel molecules, and tailoring materials with specific electronic properties, thereby bridging the gap between theoretical calculations and practical chemical synthesis.How might advances in spectroscopy techniques revolutionize our understanding of complex chemical systems?
Answer: Advances in spectroscopy, such as ultrafast laser techniques and high-resolution imaging, can capture transient intermediates and subtle changes in molecular structures. These improvements provide real-time insights into reaction dynamics, energy transfer processes, and molecular vibrations. Such detailed information can lead to breakthroughs in understanding complex biochemical pathways, material defects, and catalytic mechanisms, ultimately fostering innovations in pharmaceuticals, renewable energy, and nanotechnology.What challenges arise when modeling chemical reactions using physical chemistry principles, and how can they be overcome?
Answer: Modeling chemical reactions is challenging due to the complexity of interactions, the need for accurate computational methods, and the influence of environmental factors. Approximations in models can lead to discrepancies with experimental data. To overcome these challenges, researchers combine high-performance computing, advanced algorithms, and experimental validation to refine models. Integrating machine learning with traditional methods also shows promise in predicting reaction outcomes more reliably, bridging theory and practice.How does the concept of entropy relate to the spontaneous behavior of chemical systems?
Answer: Entropy is a measure of disorder or randomness in a system. In chemical reactions, an increase in entropy often drives spontaneity, even if the reaction is endothermic. The second law of thermodynamics states that systems tend to evolve towards greater disorder, so reactions that lead to an increase in entropy are more likely to occur spontaneously. Understanding entropy helps chemists predict reaction spontaneity and design processes that harness or control disorder for desired outcomes.What role does computational physical chemistry play in modern research and industrial applications?
Answer: Computational physical chemistry leverages computer simulations to model molecular systems, predict reaction pathways, and understand complex phenomena at the atomic level. These simulations guide experimental work, reduce trial-and-error in material design, and optimize industrial processes. In industries such as pharmaceuticals, energy, and materials science, computational tools accelerate innovation by enabling the rapid screening of compounds and the design of efficient, sustainable processes.How can understanding phase transitions lead to the development of novel materials with unique properties?
Answer: Phase transitions involve changes in the state of matter and are governed by fundamental principles of energy and molecular interactions. By controlling phase transitions—through temperature, pressure, or chemical composition—scientists can design materials that exhibit specific properties, such as superconductivity, shape memory, or enhanced strength. This understanding allows for the creation of advanced materials tailored for applications in electronics, aerospace, and biomedical devices.In what ways do external factors such as pressure and temperature influence the kinetics and thermodynamics of reactions?
Answer: Pressure and temperature are critical external factors that alter reaction rates and energy landscapes. Temperature affects kinetic energy, thus influencing reaction rates by lowering or raising the activation energy required for a reaction. Pressure, particularly in gas-phase reactions, can change concentration and collision frequency, thereby affecting both kinetics and equilibrium positions. Mastery of these variables enables chemists to fine-tune reactions for optimal yield and efficiency in various industrial and laboratory settings.How do microscopic interactions between particles manifest as macroscopic physical properties in substances?
Answer: Microscopic interactions, such as intermolecular forces and atomic bonding, dictate the macroscopic properties of substances like viscosity, conductivity, and thermal stability. For example, strong intermolecular attractions in a liquid can lead to high boiling points and surface tension, while weak interactions result in volatile substances. Understanding these interactions helps scientists predict and manipulate material properties, allowing for the engineering of substances with desired performance characteristics.What are the potential benefits and limitations of using mathematical models in predicting complex chemical behavior?
Answer: Mathematical models offer a structured approach to predicting chemical behavior by quantifying interactions and energy changes. Benefits include the ability to simulate reactions, optimize conditions, and guide experimental designs. However, limitations arise from the simplifications and approximations necessary in these models, which may not capture every nuance of real-world systems. Continuous refinement and integration with experimental data are essential to overcome these challenges and improve model accuracy.How might future innovations in physical chemistry impact renewable energy technologies?
Answer: Innovations in physical chemistry can lead to breakthroughs in renewable energy by improving the efficiency of energy conversion and storage systems. Advances in understanding catalytic processes, charge transport, and phase transitions can optimize solar cells, fuel cells, and batteries. These improvements can increase energy output, reduce costs, and enhance the sustainability of renewable technologies, contributing significantly to global energy solutions and environmental preservation.What ethical and environmental considerations should be taken into account when applying physical chemistry to industrial processes?
Answer: When applying physical chemistry in industry, it is crucial to balance innovation with sustainability and safety. Ethical considerations include minimizing environmental impact, reducing hazardous waste, and ensuring energy-efficient processes. Environmental concerns require the development of green chemistry practices that limit toxic emissions and promote renewable resources. Adopting these principles not only safeguards ecosystems and human health but also drives responsible scientific advancement and industry standards.