Fiber Optics
Fiber optics represents a transformative branch of applied physics and engineering, enabling high-speed communication, precise sensing, and minimally invasive medical procedures. At its core, it harnesses the principles of light and optics to transmit data via light signals through strands of glass or plastic fibers, often thinner than a human hair. The physical behavior of light within these fibers is governed by the laws of geometrical optics, particularly the phenomena of total internal reflection and refraction.
Fiber optics has become a fundamental part of electricity and magnetism-based systems. While no electric current flows through the fiber itself, the signals begin and end at electrical circuits, where light is converted to electrical signals and vice versa. The behavior of the light within these systems aligns with the broader understanding of electromagnetic waves, as light is one part of the electromagnetic spectrum.
A complete understanding of fiber optics also draws on electrodynamics and electromagnetic induction, particularly in the design of fiber amplifiers and signal processing units. Applications in sensor technology often depend on changes in electrostatics or magnetic fields, which alter the light’s properties as it travels through the fiber.
Modern fiber optic systems increasingly involve nonlinear phenomena, studied in nonlinear optics, where intense light interacts with the medium to generate new frequencies or switch signals. These principles are vital in advanced communication systems and ultrafast laser development, which link closely to laser optics and photonics.
In medical diagnostics, fiber optics is widely employed in endoscopes and imaging tools, forming an integral part of bio-optics. Similarly, monitoring pollution and environmental changes can involve fiber-based sensors, intersecting with atmospheric and environmental optics. These applications benefit from knowledge of visual optics when modeling the human eye or designing tools for visual inspection.
Deeper insight into fiber-based phenomena is often obtained through quantum optics, especially when designing quantum communication networks or exploring photon entanglement through optical fibers. This merges with ideas from quantum electrodynamics (QED) and modern physics, which support theoretical and technological advances.
Other specialized areas such as wave optics explain interference and diffraction effects crucial in the multiplexing of optical signals, a technique used to increase bandwidth. Research into superconducting photodetectors also draws from superconductivity, offering high-sensitivity detection for low-signal optical systems.
Even exotic plasma-based fiber designs are being studied in conjunction with plasma physics and magnetohydrodynamics (MHD) for space and defense technologies. Concepts such as magnetostatics may also be relevant when integrating magnetic control into fiber systems or sensors.
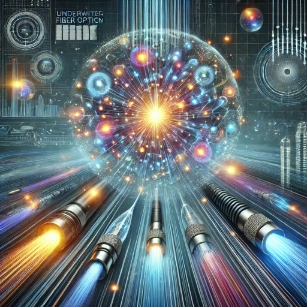
Table of Contents
Key Concepts in Fiber Optics
Structure of Optical Fibers
An optical fiber is composed of three main layers:- Core: The central region where light is transmitted. Made of high-purity glass or plastic.
- Cladding: Surrounds the core and has a lower refractive index to ensure total internal reflection.
- Coating/Buffer: A protective outer layer that shields the fiber from physical damage and moisture.
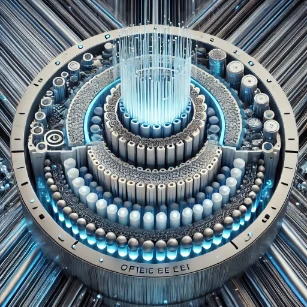
Principle of Total Internal Reflection (TIR)
Light in an optical fiber is guided by Total Internal Reflection. When light travels from a medium with a higher refractive index (core) to a lower refractive index (cladding) at an angle greater than the critical angle, it reflects entirely back into the core. Critical Angle Formula:= refractive index of the core / refractive index of the cladding
Types of Optical Fibers
Based on Mode of Propagation:
- Single-Mode Fiber (SMF):
- Core diameter: ~8-10 µm
- Transmits one mode of light, suitable for long-distance, high-bandwidth communication.
- Multi-Mode Fiber (MMF):
- Core diameter: ~50-62.5 µm
- Transmits multiple light modes, used for shorter distances due to modal dispersion.
Based on Material:
- Glass Fiber: Used for long-distance communication.
- Plastic Optical Fiber (POF): Used for short-distance applications due to higher signal loss.
Numerical Aperture (NA)
The Numerical Aperture measures a fiber’s ability to collect light.Attenuation and Dispersion
Attenuation:
Loss of signal strength as light travels through the fiber.Dispersion:
Spreading of light pulses over distance, causing signal overlap.- Modal Dispersion: Occurs in multi-mode fibers.
- Chromatic Dispersion: Different wavelengths travel at different speeds.
- Polarization Mode Dispersion (PMD): Light polarization modes travel differently.
Applications of Fiber Optics
- Telecommunications: High-speed internet, voice, and data transmission.
- Medical Imaging: Endoscopy and Optical Coherence Tomography (OCT).
- Sensing: Fiber optic sensors for temperature, strain, and pressure measurements.
- Military and Aerospace: Secure, lightweight communication systems.
- Industrial Automation: Networking and remote monitoring.
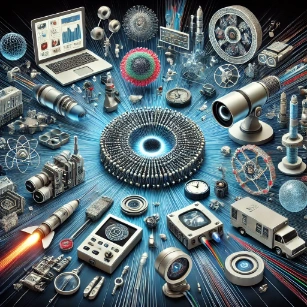
Five Numerical Examples
Example 1: Critical Angle for Total Internal Reflection
Problem: A fiber optic cable has a core refractive index of 1.48 and a cladding refractive index of 1.46. Find the critical angle for total internal reflection. Solution: Answer: The critical angle is approximately 80.57°.Example 2: Numerical Aperture of an Optical Fiber
Problem: Calculate the Numerical Aperture (NA) for a fiber with a core refractive index of 1.50 and a cladding refractive index of 1.45. Solution: Answer: The numerical aperture is 0.384.Example 3: Attenuation in Optical Fiber
Problem: If an optical signal with an input power of 10 mW is reduced to 5 mW after traveling 2 km, calculate the attenuation. Solution: Answer: The attenuation is 1.505 dB/km.Example 4: Pulse Broadening Due to Modal Dispersion
Problem: A multi-mode fiber has a modal dispersion of 0.1 ns/m. What is the pulse broadening over 2 km? Solution: Answer: The pulse broadening is 200 ns.Example 5: Maximum Acceptance Angle
Problem: Find the maximum acceptance angle for a fiber with a numerical aperture of 0.5. Solution: Answer: The maximum acceptance angle is 30°.Why Study Fiber Optic
Principles of Light Transmission in Optical Fibers
Fiber optics is the study of light transmission through thin strands of glass or plastic. Students learn about total internal reflection, numerical aperture, and attenuation. These principles explain how data can be transmitted over long distances with minimal loss. Fiber optics plays a foundational role in modern communication networks.
Telecommunications and Internet Infrastructure
Students explore how fiber optic cables enable high-speed data transfer for internet, telephone, and television services. The technology supports the backbone of global digital communication. Understanding how light is modulated and multiplexed within fibers enhances comprehension of real-time information exchange. It prepares students to contribute to telecommunications and networking industries.
Sensing and Industrial Applications
Fiber optics is also used for sensing temperature, strain, and chemical environments in industrial and environmental applications. Students study how distributed sensors provide continuous, remote monitoring. These tools are used in structural health, oil and gas, and medical diagnostics. They illustrate the wide-ranging utility of fiber-based technologies.
Design, Fabrication, and Characterization
Students gain insight into how optical fibers are manufactured and tested. They learn about single-mode vs. multimode fibers, signal dispersion, and splicing techniques. Hands-on experience builds technical skills relevant to engineering and applied physics. It fosters innovation in photonics and sensor design.
Pathway to Photonics and Optical Engineering
Studying fiber optics introduces students to broader fields such as photonics, optical communications, and signal processing. This knowledge lays the foundation for developing faster, more secure, and energy-efficient systems. It connects science with high-impact technologies. It supports careers in R&D, defense, and next-generation communication systems.
Fiber Optics: Conclusion
Fiber Optics has revolutionized global communication by enabling ultra-fast, high-capacity data transmission over vast distances with minimal loss. It relies on principles like total internal reflection, numerical aperture, and advanced light guidance mechanisms. Beyond telecommunications, fiber optics plays a pivotal role in medical imaging, sensing technologies, and industrial automation. As research continues, innovations like photonic crystal fibers and optical solitons promise even greater advancements in this transformative field.Fiber Optics: Review Questions and Answers:
1. What are optical fibers and how do they transmit light?
Answer: Optical fibers are thin strands of glass or plastic that transmit light signals over long distances using total internal reflection. Their core-cladding structure confines light, minimizing loss and enabling high-speed data transmission.
2. How does total internal reflection work in an optical fiber?
Answer: Total internal reflection occurs when light traveling in a medium with a high refractive index (the core) hits the boundary with a lower refractive index (the cladding) at an angle greater than the critical angle, causing it to reflect entirely back into the core.
3. What is the numerical aperture (NA) of an optical fiber, and why is it important?
Answer: The numerical aperture is a measure of the light-gathering ability of an optical fiber. It determines the maximum acceptance angle for light entering the fiber, affecting coupling efficiency and signal strength.
4. How does dispersion affect the performance of fiber-optic communication systems?
Answer: Dispersion causes different wavelengths of light to travel at different speeds within the fiber, leading to signal broadening and potential overlap of data pulses. This limits the bandwidth and the distance over which data can be transmitted without distortion.
5. What is attenuation in optical fibers, and what factors contribute to it?
Answer: Attenuation is the loss of light intensity as it travels through an optical fiber, caused by absorption, scattering, and bending losses. It is measured in decibels per kilometer (dB/km) and affects the overall signal quality.
6. How do single-mode and multi-mode fibers differ?
Answer: Single-mode fibers have a small core that allows only one propagation mode of light, offering higher bandwidth and longer transmission distances. Multi-mode fibers have larger cores that support multiple light paths, resulting in modal dispersion and lower bandwidth over long distances.
7. What role do optical amplifiers play in fiber-optic networks?
Answer: Optical amplifiers boost the signal strength without converting it to an electrical signal, compensating for attenuation over long distances and enabling efficient, high-speed communication in large-scale fiber-optic networks.
8. How is the refractive index profile of an optical fiber designed to minimize signal loss?
Answer: The refractive index profile is engineered so that the core has a higher refractive index than the cladding, which ensures total internal reflection. Graded-index fibers further reduce modal dispersion by gradually decreasing the refractive index from the center to the edges of the core.
9. What is the significance of the bandwidth-distance product in fiber-optic communications?
Answer: The bandwidth-distance product is a key performance metric that indicates the maximum data transmission capacity of a fiber-optic system over a given distance. A higher product means the fiber can support more data over longer distances without significant signal degradation.
10. How do fiber-optic sensors utilize light to measure environmental or structural changes?
Answer: Fiber-optic sensors detect changes in light properties—such as intensity, phase, wavelength, or polarization—as it travels through or reflects from the fiber. These changes are correlated with environmental or structural variations, enabling precise measurements for applications like temperature, strain, and pressure sensing.
Fiber Optics: Thought-Provoking Questions and Answers:
1. How might emerging photonic technologies further revolutionize fiber-optic communication systems?
Answer: Emerging photonic technologies, such as integrated photonic circuits and quantum communication, promise to dramatically increase data transmission speeds and security. These innovations could lead to ultrafast networks, reduced energy consumption, and enhanced connectivity by merging optical and electronic functions on a single chip.
2. In what ways could advances in fiber-optic materials reduce signal loss and improve transmission efficiency?
Answer: Advances in materials, including low-loss glass and novel polymers, can reduce scattering and absorption losses. Improved manufacturing techniques and material purity can enhance transmission efficiency, allowing for longer distances and higher data rates in optical networks.
3. How does the interplay between dispersion and nonlinearity affect long-haul fiber-optic communications, and what strategies can mitigate these effects?
Answer: Dispersion causes pulse broadening, while nonlinearity can distort the signal in high-power systems. Techniques like dispersion compensation, wavelength-division multiplexing, and the use of dispersion-shifted fibers help mitigate these effects, preserving signal integrity over long distances.
4. How might fiber-optic sensors contribute to advancements in smart infrastructure and environmental monitoring?
Answer: Fiber-optic sensors, due to their high sensitivity and immunity to electromagnetic interference, can be embedded in buildings, bridges, and pipelines to monitor structural health and environmental conditions in real time. This leads to proactive maintenance, improved safety, and efficient resource management.
5. What are the potential benefits and challenges of integrating fiber-optic technology with wireless communication systems?
Answer: Integrating fiber-optics with wireless systems can provide high-speed backhaul and enhanced connectivity. However, challenges include bridging the gap between optical and radio frequency domains, ensuring seamless signal conversion, and maintaining reliability in diverse environments.
6. How can polarization-maintaining fibers improve the performance of high-precision optical systems?
Answer: Polarization-maintaining fibers preserve the state of polarization of light, reducing signal degradation due to polarization mode dispersion. This is crucial in applications like interferometry, coherent communication, and high-resolution imaging, where maintaining polarization is essential for performance.
7. How might the development of optical fiber networks impact global communication infrastructure and economic growth?
Answer: Optical fiber networks offer unparalleled data transmission speeds and reliability, which are essential for modern communications. Their deployment can bridge digital divides, support the growth of internet-based economies, and drive innovation in industries such as finance, healthcare, and education, fostering global economic development.
8. In what ways do fiber-optic cables contribute to the security of communication systems?
Answer: Fiber-optic cables are difficult to tap without detection and are immune to electromagnetic interference, making them inherently secure. Their use in secure communication networks reduces the risk of data breaches and ensures the integrity of sensitive information.
9. How do advancements in fiber-optic technology influence the development of high-speed internet and data centers?
Answer: Fiber-optic technology enables the transmission of large volumes of data at high speeds with minimal loss, which is critical for high-speed internet and efficient data centers. This leads to faster download speeds, improved cloud computing services, and enhanced connectivity for businesses and consumers.
10. How might innovations in fiber-optic technology contribute to the future of telemedicine and remote diagnostics?
Answer: Advances in fiber-optic imaging and sensors allow for high-resolution, real-time data transmission from remote locations. This can enable telemedicine applications, such as remote surgeries and diagnostics, improving healthcare accessibility and patient outcomes, especially in underserved regions.
11. What are the environmental implications of expanding fiber-optic networks globally, and how can sustainability be ensured?
Answer: Expanding fiber-optic networks can reduce energy consumption compared to traditional copper networks, lower electromagnetic interference, and improve efficiency. However, considerations include the environmental impact of manufacturing and disposing of optical components. Sustainable practices in production, recycling, and energy use are essential to ensure a positive environmental impact.
12. How do advancements in fiber-optic technology pave the way for future innovations in quantum communication?
Answer: Fiber-optic networks form the backbone of quantum communication systems, enabling the transmission of quantum information over long distances. Advances in low-loss fibers, quantum repeaters, and secure encryption protocols are critical for building robust quantum networks, which could revolutionize data security and computational power.
Fiber Optics: Numerical Problems and Solutions:
1. A fiber-optic cable has a core refractive index of 1.48 and a cladding refractive index of 1.46. Calculate the numerical aperture (NA) of the fiber.
Solution:
2. If the maximum acceptance angle in air for a fiber-optic cable is 14°, what is its numerical aperture?
Solution:
3. A light source emits at 1550 nm and is coupled into an optical fiber. Calculate the frequency of the light.
Solution:
4. An optical fiber has an attenuation of 0.2 dB/km. Calculate the power loss in a 50 km fiber if the input power is 10 mW.
Solution:
Total attenuation = 0.2 dB/km x 50 km = 10 dB.
Power ratio
Output power = 10 mW x 0.1 = 1 mW
5. A single-mode fiber has a mode field diameter of 9 μm. Calculate the mode field radius in meters.
Solution:
Radius
6. A fiber-optic cable transmits data at a rate of 10 Gbps over 40 km. What is the data rate per kilometer?
Solution:
Data rate per km =
7. A fiber-optic sensor has a sensitivity of 0.5 nm/με (wavelength shift per microstrain). If the sensor measures a shift of 2 nm, calculate the strain experienced.
Solution:
Strain
8. In a fiber-optic system, if the dispersion is 17 ps/(nm·km) and the system operates over 100 km with a spectral width of 2 nm, calculate the total pulse broadening.
Solution:
Total broadening
9. A laser with a wavelength of 850 nm is coupled into a multimode fiber with a core diameter of 50 μm. If the fiber has an NA of 0.2, calculate the maximum acceptance angle in degrees.
Solution:
10. An optical amplifier boosts the signal by 20 dB. If the input power is 1 μW, what is the output power?
Solution:
20 dB corresponds to a power ratio of 100.
Output power =
11. In an optical communication system, if a fiber has a dispersion parameter of 15 ps/(nm·km) and a 5 nm spectral width is used, what is the total dispersion over 80 km?
Solution:
Total dispersion =
12. A fiber-optic cable has an effective refractive index of 1.468. Calculate the speed of light in the fiber.
Solution: