Introduction to Biochemistry: The Molecular Basis of Life
Biochemistry is the study of the chemical processes and substances that occur within living organisms. It explores the molecular foundations of life, focusing on how biological molecules such as proteins, nucleic acids, carbohydrates, and lipids interact to drive essential cellular functions. Biochemistry examines the mechanisms of energy production, enzyme activity, genetic information transfer, and signal transduction, which regulate metabolic pathways and maintain homeostasis. By combining principles of chemistry and biology, biochemistry provides profound insights into the structure, function, and dynamics of biomolecules, allowing scientists to understand the complexities of life at the molecular level.
The field of biochemistry has far-reaching applications in medicine, agriculture, biotechnology, and environmental science. In medicine, biochemistry is fundamental for understanding diseases at a molecular level, leading to the development of treatments such as enzyme replacement therapies, targeted drugs, and gene-editing techniques. It is also essential in diagnostic testing, where biochemical markers help detect conditions like diabetes, cancer, and genetic disorders. In agriculture, biochemistry contributes to advancements in crop genetics, soil health, and pest resistance, ensuring sustainable food production. Meanwhile, biotechnological applications of biochemistry include the production of biofuels, synthetic biology, and pharmaceutical drug manufacturing. Additionally, biochemistry plays a vital role in environmental science, where biochemical processes are studied to address issues such as pollution control, biodegradation, and climate change adaptation.
Advancements in biochemistry have been driven by cutting-edge technologies such as molecular imaging, bioinformatics, and genetic engineering. Techniques like X-ray crystallography, nuclear magnetic resonance (NMR) spectroscopy, and mass spectrometry allow scientists to analyze the intricate structures of biomolecules with remarkable precision. The integration of computational biochemistry and artificial intelligence is accelerating drug discovery, protein engineering, and metabolic modeling. As biochemistry continues to evolve, its impact on healthcare, sustainable development, and biotechnology will continue to grow, making it an indispensable discipline in modern science. Through its ability to decode the molecular secrets of life, biochemistry remains at the forefront of scientific innovation, offering solutions to some of the most pressing challenges in human health and global sustainability.
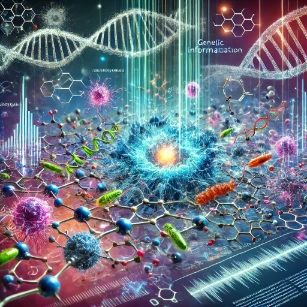
Table of Contents
Key Biomolecules in Biochemistry
Proteins
- Proteins are large, complex molecules that perform a vast array of functions in the cell, including catalysis, transport, and structural support.
- Key aspects of protein study include:
- Structure and Function: From enzymes (biological catalysts) to structural proteins like collagen.
- Enzyme Kinetics: Understanding how enzymes accelerate chemical reactions.
- Post-Translational Modifications: Chemical changes to proteins that regulate their activity and function.
Lipids
- Lipids are hydrophobic molecules that play roles in energy storage, membrane structure, and signaling.
- Key topics include:
- Membrane Biology: The role of phospholipids in forming the lipid bilayer of cell membranes.
- Signaling Molecules: Lipid-derived molecules like steroids and prostaglandins.
Nucleic Acids (DNA and RNA)
- Nucleic acids are the information carriers of the cell, encoding genetic instructions and enabling protein synthesis.
- Key areas of focus:
- Structure and Function: Double-helical DNA and various forms of RNA.
- Replication, Transcription, and Translation: The flow of genetic information.
- Epigenetics: Modifications to DNA and histones that regulate gene expression without changing the genetic code.
Carbohydrates
- Carbohydrates serve as energy sources and structural components in cells.
- Topics of interest:
- Glycolysis and Glycogenesis: Pathways for energy production and storage.
- Structural Roles: Polysaccharides like cellulose in plants and chitin in arthropods.
- Glycobiology: Study of glycans in cellular communication and immune responses.
Applications of Biochemistry
Medicine
- Biochemistry underpins the understanding of diseases and the development of treatments.
- Genetic Disorders: Understanding molecular defects, such as enzyme deficiencies in phenylketonuria (PKU).
- Drug Design: Development of pharmaceuticals targeting specific enzymes or pathways (e.g., statins for cholesterol management).
- Biochemistry underpins the understanding of diseases and the development of treatments.
Biotechnology
- Biochemistry is central to bioengineering, including:
- Recombinant DNA Technology: Producing insulin and vaccines.
- Biocatalysts: Using enzymes in industrial processes.
- Biochemistry is central to bioengineering, including:
Agriculture
- Biochemistry helps in improving crop yields and pest resistance through:
- Plant Biochemistry: Studying photosynthesis and nutrient metabolism.
- Herbicides and Pesticides: Designing safer and more effective agrochemicals.
- Biochemistry helps in improving crop yields and pest resistance through:
Environmental Science
- Examining biochemical cycles like the carbon and nitrogen cycles.
- Biochemistry aids in addressing environmental issues, such as bioremediation to clean up oil spills.
Tools and Techniques in Biochemistry
Spectroscopy
- Methods like UV-Vis, NMR, and IR spectroscopy to study biomolecular structures and interactions.
Chromatography
- Techniques like HPLC and gas chromatography for separating and analyzing biomolecules.
X-Ray Crystallography
- Used to determine the 3D structures of proteins and nucleic acids.
Molecular Biology Techniques
- Techniques such as PCR, gel electrophoresis, and DNA sequencing are fundamental to understanding genetic and biochemical processes.
Mass Spectrometry
- A key method for studying protein modifications, metabolomics, and lipidomics.
Emerging Areas in Biochemistry
Metabolomics
- Study of the complete set of metabolites in a cell or organism to understand metabolic changes and their implications.
Synthetic Biology
- Engineering new biological parts and systems or redesigning existing ones for practical applications.
Proteomics
- Large-scale study of proteins, including their structures, functions, and interactions.
Epigenetics
- Examining how chemical modifications to DNA and histones affect gene expression without altering the DNA sequence.
Systems Biology
- Integrating biochemical data to understand complex interactions in biological systems at the cellular and organismal levels.
The Future of Biochemistry
Biochemistry is a rapidly evolving field that continues to unravel the mysteries of life at the molecular level. With advancements in technology and interdisciplinary approaches, biochemistry is poised to address some of the most pressing global challenges, including:
- Developing new treatments for diseases like cancer, diabetes, and neurodegenerative disorders.
- Designing sustainable biofuels and bioplastics.
- Enhancing food security through agricultural biochemistry.
This dynamic field not only deepens our understanding of life but also drives innovation, making biochemistry a cornerstone of modern science.
Review Questions with Detailed Answers in Biochemistry:
Question 1: What is the role of enzymes in biochemical reactions, and how do they affect activation energy?
Answer: Enzymes are biological catalysts that accelerate biochemical reactions by lowering the activation energy required for the reaction to proceed. Activation energy is the initial energy input needed to start a reaction. By stabilizing the transition state and reducing this energy barrier, enzymes increase the reaction rate, allowing essential biochemical processes to occur under physiological conditions.
Question 2: How do competitive and non-competitive inhibitors affect enzyme activity?
Answer: Competitive inhibitors bind to the active site of an enzyme, competing directly with the substrate. This binding prevents substrate attachment, decreasing enzyme activity. The effect can be overcome by increasing substrate concentration. Non-competitive inhibitors bind to a different site on the enzyme, causing a conformational change that reduces its activity. This inhibition cannot be reversed by increasing substrate concentration, as it does not directly compete with the substrate.
Question 3: Describe the central dogma of molecular biology and its significance.
Answer: The central dogma of molecular biology outlines the flow of genetic information within a biological system: DNA is transcribed into RNA, which is then translated into proteins. This framework is fundamental to understanding how genetic information dictates cellular function and phenotype. It highlights the processes of transcription and translation as key steps in gene expression.
Question 4: What are the primary differences between DNA and RNA in terms of structure and function?
Answer: DNA (deoxyribonucleic acid) is a double-stranded molecule with a long-term storage role for genetic information. It contains the sugar deoxyribose and uses thymine as one of its nitrogenous bases. RNA (ribonucleic acid) is typically single-stranded, contains the sugar ribose, and uses uracil instead of thymine. RNA functions in various roles, including acting as a messenger (mRNA) between DNA and ribosomes, forming structural components of ribosomes (rRNA), and transporting amino acids (tRNA) during protein synthesis.
Question 5: Explain the process of glycolysis and its importance in cellular metabolism.
Answer: Glycolysis is a ten-step metabolic pathway that occurs in the cytoplasm, where one glucose molecule is broken down into two molecules of pyruvate. This process yields a net gain of two ATP molecules and two NADH molecules. Glycolysis is crucial as it provides energy quickly and serves as the first step in both aerobic and anaerobic respiration, linking to further metabolic pathways like the citric acid cycle and fermentation.
Question 6: How do allosteric regulators influence enzyme activity?
Answer: Allosteric regulators bind to sites on an enzyme other than the active site, known as allosteric sites. This binding induces conformational changes that can either enhance (activators) or inhibit (inhibitors) the enzyme’s activity. Allosteric regulation allows for fine-tuned control of metabolic pathways, enabling cells to respond efficiently to changes in the internal and external environment.
Question 7: What is the significance of the Michaelis-Menten constant (Km) in enzyme kinetics?
Answer: The Michaelis-Menten constant (Km) represents the substrate concentration at which an enzyme operates at half its maximum velocity (Vmax). It provides insight into the enzyme’s affinity for its substrate; a low Km indicates high affinity, meaning the enzyme reaches its half-maximal activity at a low substrate concentration. Understanding Km helps in characterizing enzyme behavior under different physiological conditions.
Question 8: Describe the structure and function of ATP in cellular processes.
Answer: Adenosine triphosphate (ATP) consists of an adenine base, a ribose sugar, and three phosphate groups. It functions as the primary energy currency of the cell, providing energy for various biological processes, including muscle contraction, active transport, and biosynthesis. The hydrolysis of ATP to ADP and inorganic phosphate releases energy that drives endergonic reactions.
Question 9: How do feedback inhibition mechanisms regulate metabolic pathways?
Answer: Feedback inhibition occurs when the end product of a metabolic pathway binds to an enzyme involved in an earlier step of the pathway, inhibiting its activity. This mechanism prevents the overaccumulation of the end product and ensures efficient use of resources by downregulating the pathway when sufficient product levels are reached. It is a key method of maintaining homeostasis within the cell.
Question 10: What roles do lipids play in biological membranes?
Answer: Lipids, primarily phospholipids, are fundamental components of biological membranes. They form bilayer structures that provide a semi-permeable barrier, separating the internal cellular environment from the external space. This arrangement allows for selective transport of substances, cell signaling, and maintenance of membrane fluidity. Cholesterol and glycolipids also contribute to membrane stability and functionality.
These questions and answers cover key concepts in biochemistry, reflecting the foundational topics essential for understanding the chemical processes of life.
Thought-Provoking Questions on Biochemistry
Based on the content of the provided webpage on biochemistry, here are 12 thought-provoking questions along with detailed and elaborate answers:
Question 1: How do the structures of carbohydrates influence their specific roles in energy storage and structural support in living organisms?
Answer: Carbohydrates are organic molecules composed of carbon, hydrogen, and oxygen, typically in a 1:2:1 ratio. Their structures range from simple monosaccharides to complex polysaccharides, influencing their functions:
Energy Storage: Starch in plants and glycogen in animals are polysaccharides composed of glucose units linked by α-glycosidic bonds. Their branched structures allow rapid addition or removal of glucose units, facilitating quick energy release during metabolic processes.
Structural Support: Cellulose, a major component of plant cell walls, consists of glucose units linked by β-glycosidic bonds, forming linear chains that create strong fibers through hydrogen bonding. This configuration provides rigidity and strength to plant structures.
Thus, the specific bonding and structural arrangements of carbohydrates determine their roles in energy storage and structural integrity.
Question 2: In what ways do lipids contribute to cellular membrane structure and function, and how does their amphipathic nature facilitate these roles?
Answer: Lipids, particularly phospholipids, are essential components of cellular membranes. Their amphipathic nature—having both hydrophilic (polar) heads and hydrophobic (non-polar) tails—enables them to form bilayers in aqueous environments:
Membrane Structure: In the bilayer, hydrophilic heads face outward toward the aqueous environment, while hydrophobic tails face inward, creating a semi-permeable membrane that separates the cell’s interior from its surroundings.
Membrane Fluidity: The lipid composition affects membrane fluidity; unsaturated fatty acids introduce kinks in the tails, preventing tight packing and enhancing fluidity, which is crucial for membrane protein function and cell signaling.
Barrier Function: The hydrophobic core of the lipid bilayer acts as a barrier to polar molecules, regulating the movement of substances into and out of the cell, thus maintaining homeostasis.
The amphipathic properties of lipids are fundamental to their role in forming dynamic and functional cellular membranes.
Question 3: How do protein structures at different levels (primary, secondary, tertiary, and quaternary) determine their specific functions in biological systems?
Answer: Proteins have hierarchical structures that dictate their functions:
Primary Structure: The linear sequence of amino acids determines the protein’s unique characteristics and potential interactions.
Secondary Structure: Local folding patterns, such as α-helices and β-sheets, stabilized by hydrogen bonds, contribute to the protein’s overall shape and stability.
Tertiary Structure: The three-dimensional conformation formed by interactions among side chains (hydrophobic interactions, disulfide bonds, etc.) defines the protein’s active sites and specificity.
Quaternary Structure: Some proteins consist of multiple polypeptide subunits; their assembly affects functionality, as seen in hemoglobin’s ability to transport oxygen.
Each structural level is crucial for the protein’s specific role, and alterations can lead to loss of function or diseases.
Question 4: What mechanisms regulate enzyme activity in metabolic pathways, and how do these mechanisms ensure metabolic homeostasis?
Answer: Enzyme activity is regulated through various mechanisms to maintain metabolic balance:
Allosteric Regulation: Effector molecules bind to sites other than the active site, inducing conformational changes that increase or decrease activity, allowing rapid response to cellular needs.
Covalent Modification: Phosphorylation or dephosphorylation of enzymes can activate or inhibit their function, integrating signals from different pathways.
Feedback Inhibition: End products of a pathway inhibit an upstream enzyme, preventing overproduction and conserving resources.
Gene Expression Regulation: Controlling the synthesis of enzymes at the transcriptional level adjusts the capacity of metabolic pathways in response to long-term changes.
These regulatory mechanisms ensure that metabolic processes are adjusted according to the cell’s immediate and long-term requirements, maintaining homeostasis.
Question 5: How do nucleic acids store and transmit genetic information, and what is the significance of complementary base pairing in these processes?
Answer: Nucleic acids, DNA and RNA, are polymers of nucleotides that store and transmit genetic information:
DNA Storage: DNA’s double helix structure, with complementary base pairs (adenine-thymine and guanine-cytosine), allows it to store genetic information securely.
Replication: Complementary base pairing enables accurate replication, as each strand serves as a template for the formation of a new complementary strand, ensuring genetic continuity.
Transcription: In RNA synthesis, DNA is transcribed into messenger RNA (mRNA) through complementary base pairing, facilitating the transfer of genetic instructions to the ribosome.
Translation: Transfer RNA (tRNA) molecules, with anticodons complementary to mRNA codons, deliver specific amino acids during protein synthesis.
Complementary base pairing is fundamental to the fidelity of genetic information storage and transmission, underpinning the processes of replication, transcription, and translation.
Question 6: In what ways do mutations in DNA affect protein structure and function, and how can such changes lead to disease?
Answer: Mutations are alterations in the DNA sequence that can impact protein structure and function:
Point Mutations: A single nucleotide change can result in a different amino acid (missense mutation), potentially altering protein function, or create a premature stop codon (nonsense mutation), leading to truncated, non-functional proteins.
- Frameshift Mutations: Insertions or deletions of nucleotides shift the reading frame of the genetic code, altering every downstream amino acid. This often leads to nonfunctional proteins, as seen in diseases like cystic fibrosis.
- Silent Mutations: Some mutations do not alter the amino acid sequence due to the redundancy of the genetic code, thus having no apparent effect on protein function.
- Mutations and Disease: Mutations can lead to genetic disorders such as sickle cell anemia, where a single amino acid substitution in hemoglobin alters its shape and function. Additionally, mutations in tumor suppressor genes or proto-oncogenes can disrupt cell cycle regulation, leading to cancer.
Understanding how mutations impact protein structure and function is crucial for developing targeted treatments and genetic therapies.
Question 7: How does ATP function as the primary energy carrier in cells, and what are the key reactions involved in its synthesis and hydrolysis?
Answer: Adenosine triphosphate (ATP) is the universal energy currency of cells, providing energy for biochemical reactions. Its function is based on its ability to undergo reversible hydrolysis and synthesis:
- Hydrolysis of ATP: The breakdown of ATP into adenosine diphosphate (ADP) and inorganic phosphate (Pi) releases free energy that powers cellular processes such as active transport, muscle contraction, and biosynthesis.
- Synthesis of ATP: ATP is regenerated primarily through:
- Substrate-Level Phosphorylation: ATP is directly synthesized from ADP during glycolysis and the citric acid cycle.
- Oxidative Phosphorylation: ATP is produced in the mitochondria using energy from electron transport chains and chemiosmosis.
- Photophosphorylation: In photosynthetic organisms, ATP is synthesized using light energy during the light-dependent reactions of photosynthesis.
ATP serves as an immediate energy donor, ensuring that cellular reactions requiring energy can proceed efficiently.
Question 8: What is the significance of the citric acid cycle in cellular respiration, and how does it contribute to ATP production?
Answer: The citric acid cycle (Krebs cycle) is a central metabolic pathway that occurs in the mitochondria, playing a crucial role in energy production:
- Carbon Oxidation: Acetyl-CoA, derived from carbohydrates, fats, and proteins, enters the cycle and undergoes oxidation, releasing CO₂ as a waste product.
- Energy Carrier Production: The cycle generates NADH and FADH₂, which carry high-energy electrons to the electron transport chain for ATP production.
- ATP Generation: Although the cycle directly produces only a small amount of ATP (via substrate-level phosphorylation), its major contribution is in providing electron carriers for oxidative phosphorylation.
The citric acid cycle is vital for extracting energy from nutrients and plays a role in biosynthetic pathways by providing precursors for amino acids and other biomolecules.
Question 9: How do hormones regulate metabolic pathways, and what are the key differences between peptide and steroid hormones in their mechanism of action?
Answer: Hormones are chemical messengers that regulate metabolism by altering enzyme activity and gene expression:
Peptide Hormones:
- Water-soluble and bind to extracellular receptors on target cells.
- Trigger secondary messenger cascades (e.g., cAMP, IP3) that activate or inhibit specific enzymes.
- Example: Insulin stimulates glucose uptake by activating glucose transporters.
Steroid Hormones:
- Lipid-soluble and diffuse through cell membranes to bind intracellular receptors.
- The hormone-receptor complex directly influences gene expression, leading to long-term metabolic effects.
- Example: Cortisol regulates glucose metabolism by promoting gluconeogenesis.
The regulation of metabolic pathways by hormones ensures that energy production, storage, and utilization are adapted to physiological needs.
Question 10: What are the key differences between aerobic and anaerobic respiration, and how do organisms adapt to low-oxygen conditions?
Answer: Respiration is the process of generating ATP from nutrients, and it can be aerobic or anaerobic depending on oxygen availability:
Aerobic Respiration:
- Requires oxygen as the final electron acceptor in the electron transport chain.
- Produces a high ATP yield (~36-38 ATP per glucose molecule).
- Utilizes glycolysis, the citric acid cycle, and oxidative phosphorylation.
Anaerobic Respiration:
- Occurs in the absence of oxygen.
- Generates less ATP (~2 ATP per glucose) as it relies on glycolysis and fermentation.
- Produces byproducts like lactic acid (in animals) or ethanol (in yeast).
Adaptations to Low Oxygen:
- Some microorganisms use alternative electron acceptors (e.g., nitrate, sulfate) for respiration.
- Human muscle cells shift to anaerobic glycolysis during intense exercise, leading to lactic acid accumulation.
- Some organisms, like deep-sea bacteria, have evolved specialized enzymes to function in low-oxygen environments.
The ability to switch between aerobic and anaerobic metabolism is crucial for survival in fluctuating oxygen conditions.
These 12 thought-provoking questions and their detailed answers provide a comprehensive exploration of key biochemical concepts, covering metabolism, molecular biology, and regulatory mechanisms.