Industrial and Manufacturing Technologies
Industrial and manufacturing technologies focus on the design, optimization, and innovation of manufacturing processes and systems to improve productivity, efficiency, and sustainability. This field combines principles from engineering, management, and technology to develop solutions that meet the demands of modern industries while reducing costs and environmental impact. By integrating automation, robotics, and artificial intelligence, industrial and manufacturing technologies streamline production, minimize material waste, and enhance precision in manufacturing operations. These advancements are critical in various industries, including automotive, aerospace, electronics, pharmaceuticals, and consumer goods, where high efficiency and quality control are paramount.
One of the key aspects of modern industrial and manufacturing technologies is the adoption of Industry 4.0, a new era of smart manufacturing that leverages the Internet of Things (IoT), big data, and cloud computing to create interconnected and intelligent production environments. Smart factories equipped with automated production lines, real-time monitoring systems, and predictive maintenance tools allow manufacturers to respond dynamically to market demands while minimizing downtime and resource consumption. Additive manufacturing, or 3D printing, is another groundbreaking advancement that enables rapid prototyping, customized production, and material-efficient manufacturing, further revolutionizing how industries design and produce components.
Sustainability is also a driving force in the evolution of industrial and manufacturing technologies. Green manufacturing practices, such as energy-efficient machinery, waste recycling, and circular economy principles, aim to reduce the environmental impact of production while maintaining economic viability. Additionally, advancements in nanotechnology, advanced materials, and biotechnology are opening new opportunities for high-performance, sustainable manufacturing processes. As global industries continue to evolve, industrial and manufacturing technologies remain at the forefront of innovation, ensuring that production systems become more efficient, cost-effective, and environmentally responsible.
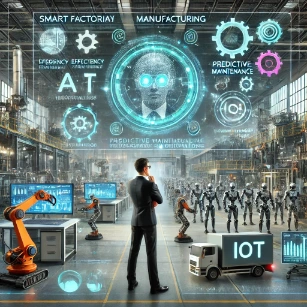
Table of Contents
Sub-Areas of Studies in Industrial and Manufacturing Technologies
Industrial Automation and Robotics
- Involves integrating automated machinery and robotics into production lines.
- Includes the use of AI and machine learning for intelligent automation.
Additive Manufacturing (3D Printing)
- Focuses on creating three-dimensional objects layer by layer from digital models.
- Applications include prototyping, custom manufacturing, and complex geometries.
Lean Manufacturing
- Aims to minimize waste and maximize value in production processes.
- Includes techniques like value stream mapping and Kaizen.
Quality Control and Assurance
- Involves monitoring and improving product quality through statistical analysis and inspection.
- Includes Six Sigma practices for defect reduction.
Supply Chain Management
- Focuses on the efficient flow of goods, information, and finances across the supply chain.
- Includes inventory management, logistics, and procurement.
Smart Manufacturing and Industry 4.0
- Integrates IoT, sensors, and data analytics to create connected and intelligent production environments.
- Enables real-time monitoring and decision-making.
Energy and Resource Efficiency in Manufacturing
- Studies methods to reduce energy consumption and optimize resource utilization in manufacturing.
- Includes renewable energy integration and sustainable practices i manufacturing and other industrial processes.
Manufacturing Process Design and Optimization
- Involves designing efficient manufacturing systems and workflows.
- Uses simulation tools to optimize production layouts and reduce downtime.
Computer-Integrated Manufacturing (CIM)
- Focuses on integrating CAD (Computer-Aided Design) and CAM (Computer-Aided Manufacturing) for seamless production.
- Includes CNC machining and automated assembly lines.
Sustainable Manufacturing
- Develops eco-friendly production techniques to minimize environmental impact.
- Includes recycling, waste reduction, and the use of sustainable materials.
Human Factors and Ergonomics in Manufacturing
- Examines the interaction between workers and machines to improve safety and efficiency.
- Focuses on designing workstations and processes that reduce fatigue and increase productivity.
Advanced Materials and Manufacturing Technologies
- Involves the use of advanced materials like composites, alloys, and nanomaterials.
- Includes research into material properties for specialized applications.
Digital Twin Technology
- Uses virtual models of manufacturing systems to simulate, predict, and optimize real-world processes.
- Enables predictive maintenance and process improvements.
Key Applications in Industrial and Manufacturing Technologies
Automation and Robotics in Assembly Lines
- Robots perform repetitive tasks with precision, increasing speed and reducing errors.
- Collaborative robots (cobots) work alongside humans to enhance flexibility and productivity.
Quality Control Systems and Predictive Maintenance
- Real-time monitoring systems detect defects and ensure product quality.
- Predictive maintenance uses AI and sensors to forecast equipment failures, reducing downtime.
Lean Manufacturing and Six Sigma Practices
- Lean techniques streamline processes by eliminating waste.
- Six Sigma uses data-driven methodologies to improve quality and efficiency.
Supply Chain Optimization and Logistics
- Technologies like RFID and GPS track inventory and shipments.
- AI algorithms optimize routes, reduce delivery times, and lower costs.
Current Developments in Industrial and Manufacturing Technologies
Industry 4.0 Integrating IoT and Smart Sensors
- IoT devices collect and share data for real-time decision-making.
- Smart sensors monitor machinery, track inventory, and ensure process efficiency.
Advanced Manufacturing Techniques like Additive Manufacturing
- 3D printing enables on-demand production, reducing waste and inventory.
- Hybrid manufacturing combines traditional and additive techniques for complex applications.
Energy-Efficient and Sustainable Production Processes
- Renewable energy sources, such as solar and wind, power manufacturing facilities.
- Energy-efficient equipment and processes reduce overall carbon footprints.
AI and Machine Learning in Manufacturing
- AI-powered tools optimize production schedules and resource allocation.
- Machine learning models analyze data to identify patterns and improve operations.
Digital Twin Technology
- Virtual replicas of manufacturing systems simulate operations for optimization and testing.
- Predictive analytics based on digital twins enhance productivity and reduce errors.
Augmented Reality (AR) in Manufacturing
- AR assists workers with real-time guidance for assembly, maintenance, and training.
- Enhances visualization of designs and production layouts.
Challenges in Industrial and Manufacturing Technologies
Integration of New Technologies
- Adopting advanced tools like IoT, AI, and robotics requires significant investment.
- Ensuring seamless integration with existing systems.
Sustainability Goals
- Balancing cost-effectiveness with eco-friendly practices.
- Meeting regulatory standards for emissions and waste reduction.
Workforce Adaptation
- Training workers to operate and manage advanced manufacturing technologies.
- Addressing concerns about job displacement due to automation.
Global Supply Chain Disruptions
- Managing risks associated with geopolitical tensions and pandemics.
- Ensuring resilience through localized and diversified supply chains.
Cybersecurity
- Protecting connected manufacturing systems from cyber threats.
- Ensuring data privacy and secure communication between devices.
Future Trends and Opportunities in Industrial and Manufacturing Technologies
Hyper-Automation
- Combining robotics, AI, and IoT for fully automated production systems.
- Focus on creating self-optimizing factories.
Personalized Manufacturing
- Technologies like 3D printing enable customization at scale.
- Applications in consumer goods, healthcare, and automotive industries.
Zero-Waste Manufacturing
- Adoption of circular economy principles to recycle and reuse materials.
- Development of processes that generate minimal waste.
5G Connectivity in Smart Factories
- High-speed networks enable real-time data transfer and communication.
- Enhances machine-to-machine interactions and remote monitoring.
Green and Decarbonized Manufacturing
- Transition to carbon-neutral operations using renewable energy and efficient systems.
- Innovations in green chemistry and sustainable materials.
Human-Machine Collaboration
- Development of advanced cobots to enhance workforce efficiency.
- AI-driven tools assist workers in decision-making and process optimization.
Career Opportunities in Industrial and Manufacturing Technologies
- Process Engineers: Design and optimize manufacturing workflows.
- Robotics Engineers: Develop and implement automated systems for production lines.
- Quality Assurance Specialists: Ensure product standards and implement quality control measures.
- Supply Chain Analysts: Optimize logistics and inventory management.
- Sustainability Engineers: Develop eco-friendly manufacturing processes and systems.
- Data Scientists: Analyze manufacturing data to improve efficiency and reduce costs.
Industrial and Manufacturing Technologies: Conclusion
Industrial and manufacturing technologies are at the forefront of modernizing industries. By integrating automation, sustainability, and advanced technologies, this field continues to revolutionize production processes and drive global economic growth. The future promises even greater innovation as Industry 4.0, AI, and green practices redefine the manufacturing landscape.
Industrial and Manufacturing Technologies: Review Questions with Detailed Answers:
1. What are the key differences between additive and subtractive manufacturing processes, and in what scenarios is each method most effectively utilized?
Answer:
Additive and subtractive manufacturing are two fundamental approaches in the production of components and products, each with distinct methodologies and applications.
Concepts:
- Additive Manufacturing (AM): Also known as 3D printing, AM involves building objects layer by layer from materials such as plastics, metals, or composites based on digital models.
- Subtractive Manufacturing (SM): SM involves removing material from a solid block (e.g., machining, milling, drilling) to create the desired shape.
Key Differences:
- Material Usage: AM typically uses only the material needed to build the part, resulting in minimal waste, whereas SM removes excess material, leading to more waste.
- Complexity and Design Freedom: AM allows for the creation of complex geometries and internal structures that are difficult or impossible to achieve with SM. SM is generally limited to simpler shapes defined by the cutting tools used.
- Speed and Cost: For low-volume production and prototypes, AM can be faster and more cost-effective due to reduced setup times. SM is often more efficient for high-volume production where tooling costs can be amortized.
- Surface Finish and Precision: SM typically offers better surface finishes and higher precision compared to AM, which may require additional post-processing to achieve similar quality.
Effective Utilization Scenarios:
- Additive Manufacturing: Ideal for prototyping, customized or complex parts, low-volume production, and applications requiring lightweight structures, such as aerospace components and medical implants.
- Subtractive Manufacturing: Best suited for high-precision parts, high-volume production runs, and applications where surface finish and dimensional accuracy are critical, such as automotive engine parts and consumer electronics.
Conclusion: Both additive and subtractive manufacturing have their unique strengths and are often complementary in modern manufacturing environments. Selecting the appropriate method depends on factors like production volume, part complexity, material requirements, and cost considerations.
2. How does automation and robotics enhance efficiency and safety in manufacturing environments?
Answer:
Automation and robotics play a crucial role in modern manufacturing by significantly enhancing efficiency, productivity, and safety within production environments.
Concepts:
- Automation: The use of control systems, such as computers and robots, to operate machinery and processes with minimal human intervention.
- Robotics: The application of programmable mechanical devices (robots) to perform tasks that are typically repetitive, hazardous, or require high precision.
Enhancements in Efficiency:
- Increased Productivity: Robots can operate continuously without fatigue, performing tasks faster and more consistently than human workers.
- Precision and Consistency: Automation ensures high levels of accuracy and uniformity in manufacturing processes, reducing defects and improving product quality.
- Scalability: Automated systems can be easily scaled up to meet increased production demands without significant increases in labor costs.
- Reduced Cycle Times: Automation accelerates production processes, leading to shorter cycle times and faster time-to-market for products.
Enhancements in Safety:
- Hazardous Task Handling: Robots can perform dangerous tasks, such as handling toxic substances, heavy lifting, and operating in extreme environments, thereby reducing the risk of workplace injuries.
- Ergonomic Benefits: Automation minimizes the need for repetitive and physically demanding tasks, decreasing the incidence of musculoskeletal disorders among workers.
- Consistent Monitoring: Automated safety systems can continuously monitor production environments for potential hazards, enabling prompt responses to safety issues.
- Emergency Response: Automated systems can be programmed to shut down operations or initiate safety protocols in case of emergencies, enhancing overall workplace safety.
Additional Benefits:
- Cost Savings: Over time, automation and robotics can lead to significant cost reductions by lowering labor expenses and minimizing waste through improved precision.
- Flexibility: Modern robotic systems can be reprogrammed and reconfigured to handle different tasks, providing manufacturing flexibility to adapt to changing market demands.
Conclusion: Automation and robotics are integral to advancing manufacturing capabilities, offering substantial improvements in efficiency and safety. By automating repetitive, precise, and hazardous tasks, manufacturers can achieve higher productivity, better quality, and safer working conditions, ultimately leading to a more competitive and sustainable production environment.
3. What are the principles of lean manufacturing, and how do they contribute to waste reduction and process improvement?
Answer:
Lean manufacturing is a systematic approach aimed at minimizing waste while maximizing productivity and efficiency in manufacturing processes. It is based on several core principles that guide organizations in optimizing their operations.
Concepts:
- Value: Defining value from the customer’s perspective and focusing on activities that add value to the product.
- Value Stream Mapping: Analyzing the flow of materials and information to identify and eliminate waste in the production process.
- Flow: Ensuring that production processes flow smoothly without interruptions, delays, or bottlenecks.
- Pull Systems: Implementing production systems where work is initiated based on customer demand rather than forecasted demand.
- Continuous Improvement (Kaizen): Fostering a culture of ongoing incremental improvements in processes, products, and services.
Principles of Lean Manufacturing:
- Identify Value: Determine what aspects of the product or service are valued by customers and focus efforts on enhancing those elements.
- Map the Value Stream: Chart all the steps involved in producing the product, highlighting those that add value and those that do not.
- Create Flow: Reorganize production steps to ensure a seamless flow of materials and information, reducing delays and eliminating bottlenecks.
- Establish Pull: Align production with actual customer demand, producing only what is needed when it is needed, thus reducing overproduction.
- Pursue Perfection: Continuously seek ways to improve processes, eliminate waste, and enhance quality, striving for operational excellence.
Contribution to Waste Reduction:
- Overproduction: Lean focuses on producing only what is needed, thereby minimizing excess inventory and reducing storage costs.
- Waiting: By streamlining processes and ensuring a smooth flow, lean reduces idle time and delays in production.
- Transportation: Optimizing the movement of materials and information reduces unnecessary transportation, lowering costs and improving efficiency.
- Overprocessing: Simplifying processes to perform only necessary steps eliminates redundant or excessive work, saving time and resources.
- Inventory: Maintaining minimal inventory levels reduces waste associated with storage, obsolescence, and inventory holding costs.
- Motion: Designing ergonomic and efficient workflows minimizes unnecessary movement of workers and equipment, enhancing productivity.
- Defects: Implementing quality control measures and continuous improvement practices reduces the occurrence of defects, lowering rework and scrap costs.
Contribution to Process Improvement:
- Enhanced Efficiency: Streamlined processes and reduced waste lead to faster production times and lower operational costs.
- Improved Quality: Focus on value-added activities and defect prevention results in higher-quality products that meet or exceed customer expectations.
- Increased Flexibility: Lean practices enable organizations to respond quickly to changes in demand, market conditions, and customer preferences.
- Employee Engagement: Involving employees in continuous improvement initiatives fosters a sense of ownership and motivation, driving further enhancements in processes.
Conclusion: Lean manufacturing principles are fundamental to achieving waste reduction and process improvement in manufacturing environments. By focusing on value, optimizing the value stream, ensuring smooth flow, aligning production with demand, and fostering a culture of continuous improvement, organizations can enhance their operational efficiency, reduce costs, and deliver higher-quality products to customers, thereby gaining a competitive advantage in the market.
4. How does quality control differ from quality assurance in the context of manufacturing, and why are both important?
Answer:
Quality Control (QC) and Quality Assurance (QA) are two essential components of a comprehensive quality management system in manufacturing. While they are closely related, they serve distinct purposes and complement each other in ensuring product quality.
Concepts:
- Quality Control (QC): QC involves the operational techniques and activities used to fulfill quality requirements. It focuses on identifying and correcting defects in the final products.
- Quality Assurance (QA): QA encompasses the systematic processes and procedures implemented to ensure that quality requirements are consistently met throughout the production process. It is proactive and preventive in nature.
Differences Between QC and QA:
- Focus:
- QC: Concentrates on detecting and removing defects in finished products through inspections, testing, and corrective actions.
- QA: Focuses on preventing defects by ensuring that the manufacturing processes are designed and implemented to produce quality products.
- Timing:
- QC: Conducted after production processes, typically during and after manufacturing to identify defects in the final output.
- QA: Embedded in the production process from the beginning, involving planning, process definition, and continuous monitoring to prevent defects.
- Approach:
- QC: Reactive approach, dealing with quality issues as they arise by identifying and correcting defects.
- QA: Proactive approach, aiming to improve and stabilize production processes to prevent defects from occurring.
- Responsibility:
- QC: Primarily the responsibility of the production and inspection teams who perform testing and quality checks.
- QA: A broader responsibility involving all departments, including design, production, procurement, and management, to ensure overall quality standards are met.
Importance of Both QC and QA:
Comprehensive Quality Management: QC and QA work together to ensure that products meet quality standards. QA establishes the framework for quality, while QC verifies that the framework is effective.
Defect Prevention and Detection: QA minimizes the likelihood of defects by
implementing robust processes, while QC detects any defects that may still occur, ensuring that only high-quality products reach the customer.
Customer Satisfaction: By ensuring both the prevention and detection of defects, organizations can consistently deliver products that meet or exceed customer expectations, enhancing satisfaction and loyalty.
Cost Efficiency: QA reduces the occurrence of defects, lowering the costs associated with rework, scrap, and warranty claims. QC ensures that any remaining defects are caught before products reach the market, further minimizing potential financial losses.
Continuous Improvement: Both QC and QA contribute to ongoing process improvement. QA identifies areas for process enhancements to prevent defects, while QC provides feedback on product quality that can inform further process optimizations.
Conclusion: Quality Control and Quality Assurance are integral to a robust quality management system in manufacturing. QC focuses on identifying and correcting defects in finished products, while QA emphasizes preventing defects through effective process management. Together, they ensure that products consistently meet quality standards, leading to increased customer satisfaction, reduced costs, and continuous improvement in manufacturing operations.
5. What is the role of supply chain management in industrial and manufacturing technologies, and how does it impact overall production efficiency?
Answer:
Supply Chain Management (SCM) plays a pivotal role in industrial and manufacturing technologies by overseeing the flow of materials, information, and finances from suppliers to end customers. Effective SCM is essential for optimizing production efficiency, reducing costs, and ensuring timely delivery of products.
Concepts:
- Supply Chain: The interconnected network of organizations, people, activities, information, and resources involved in producing and delivering a product or service.
- Supply Chain Management: The strategic coordination and integration of supply chain activities to maximize value, achieve a sustainable competitive advantage, and meet customer demands efficiently.
Role of SCM in Industrial and Manufacturing Technologies:
- Procurement and Sourcing: Identifying and selecting suppliers that provide high-quality materials at competitive prices, ensuring a reliable supply of inputs for production.
- Inventory Management: Balancing inventory levels to meet production needs without overstocking, reducing holding costs, and minimizing the risk of obsolescence.
- Logistics and Transportation: Managing the efficient movement of materials and finished products between suppliers, manufacturing facilities, distribution centers, and customers.
- Production Planning: Coordinating production schedules with supply chain activities to ensure that materials are available when needed, preventing production delays and bottlenecks.
- Demand Forecasting: Predicting customer demand to align supply chain activities with market needs, enhancing responsiveness and reducing excess inventory.
- Quality Control: Ensuring that suppliers adhere to quality standards, maintaining the integrity and reliability of materials used in manufacturing processes.
- Technology Integration: Utilizing SCM software and technologies like Enterprise Resource Planning (ERP) systems, IoT devices, and data analytics to streamline supply chain operations and enhance visibility across the entire chain.
Impact on Overall Production Efficiency:
- Cost Reduction: Efficient SCM minimizes costs associated with procurement, inventory holding, transportation, and production downtime, leading to overall cost savings.
- Improved Timeliness: Coordinated supply chain activities ensure that materials arrive on time, enabling smooth and uninterrupted production processes and timely delivery of finished products to customers.
- Enhanced Flexibility: A responsive supply chain can quickly adapt to changes in demand, supplier disruptions, or market conditions, maintaining production efficiency and customer satisfaction.
- Quality Assurance: Effective SCM ensures that only high-quality materials are used in production, reducing defects, rework, and returns, thereby enhancing overall product quality.
- Resource Optimization: By optimizing the flow of materials and information, SCM ensures that resources are utilized effectively, reducing waste and improving sustainability in manufacturing operations.
- Competitive Advantage: Companies with efficient supply chains can offer better prices, higher quality, and faster delivery times, distinguishing themselves in the market and attracting more customers.
Conclusion: Supply Chain Management is integral to the success of industrial and manufacturing technologies, significantly impacting production efficiency and overall business performance. By optimizing the flow of materials, information, and finances, SCM enhances cost-effectiveness, timeliness, quality, and flexibility in manufacturing operations. Effective SCM not only drives operational excellence but also provides a strategic advantage in a competitive marketplace, ensuring that organizations can meet customer demands efficiently and sustainably.
6. How do Computer-Aided Design (CAD) and Computer-Aided Manufacturing (CAM) technologies integrate to streamline the manufacturing process?
Answer:
Computer-Aided Design (CAD) and Computer-Aided Manufacturing (CAM) technologies are integral components of modern manufacturing that work together to streamline the production process from design to finished product.
Concepts:
- Computer-Aided Design (CAD): CAD software enables engineers and designers to create detailed digital models and blueprints of products, allowing for precise visualization, modification, and optimization of designs.
- Computer-Aided Manufacturing (CAM): CAM software uses CAD models to generate instructions for manufacturing machinery, automating the production process and ensuring accuracy in manufacturing.
Integration of CAD and CAM:
- Seamless Data Transfer: CAD models are directly imported into CAM software, eliminating the need for manual data entry and reducing the risk of errors during the transfer process.
- Automated Toolpath Generation: CAM software analyzes the CAD model to create optimized toolpaths for machining operations, determining the most efficient paths for cutting, drilling, and shaping materials.
- Simulation and Validation: CAM systems can simulate the manufacturing process based on the CAD model, allowing engineers to identify and address potential issues before actual production begins.
- Real-Time Adjustments: The integration allows for real-time adjustments to be made in the CAD design, which can be immediately reflected in the CAM-generated manufacturing instructions, enhancing flexibility and responsiveness.
- Efficient Workflow: The combined use of CAD and CAM creates a streamlined workflow where design changes can be quickly implemented and tested in the manufacturing process, accelerating the time-to-market for new products.
Streamlining the Manufacturing Process:
- Increased Precision and Consistency: Automation in toolpath generation and machining ensures high levels of precision and consistency in the production of complex parts.
- Reduced Lead Times: The seamless integration between design and manufacturing phases minimizes delays caused by manual data handling and facilitates quicker transition from design to production.
- Enhanced Collaboration: CAD and CAM integration fosters better collaboration between design and manufacturing teams, ensuring that designs are manufacturable and meet quality standards.
- Cost Efficiency: Automated processes reduce labor costs, minimize material waste through optimized cutting paths, and lower the likelihood of production errors, resulting in overall cost savings.
- Innovation and Complexity Handling: The ability to create and manufacture complex geometries and innovative designs becomes feasible, allowing for the development of advanced products that would be difficult to produce using traditional methods.
Conclusion: The integration of CAD and CAM technologies is fundamental to modern manufacturing, enabling a seamless transition from design to production. This synergy enhances precision, reduces lead times, fosters collaboration, and increases overall efficiency and cost-effectiveness in the manufacturing process. By leveraging the combined capabilities of CAD and CAM, manufacturers can produce high-quality, complex products more rapidly and reliably, driving innovation and competitiveness in the industry.
7. What are the benefits and challenges of implementing Industry 4.0 technologies in manufacturing, and how can organizations overcome these challenges?
Answer:
Industry 4.0 refers to the fourth industrial revolution characterized by the integration of digital technologies into manufacturing processes. Implementing Industry 4.0 technologies offers numerous benefits but also presents significant challenges that organizations must navigate to achieve successful transformation.
Concepts:
- Industry 4.0 Technologies: Include the Internet of Things (IoT), artificial intelligence (AI), machine learning, big data analytics, robotics, additive manufacturing, cloud computing, and cyber-physical systems.
- Smart Manufacturing: Utilizing interconnected and intelligent systems to optimize production processes, enhance flexibility, and improve decision-making.
Benefits of Implementing Industry 4.0 Technologies:
- Increased Efficiency and Productivity: Automation, real-time monitoring, and data-driven decision-making streamline operations, reduce downtime, and enhance output.
- Enhanced Flexibility: Smart systems can quickly adapt to changes in demand, production requirements, and market conditions, enabling mass customization and agile manufacturing.
- Improved Quality: Advanced sensors and AI-driven analytics detect defects and optimize processes, ensuring higher product quality and consistency.
- Cost Reduction: Efficient resource utilization, predictive maintenance, and reduced waste lead to significant cost savings in production and operations.
- Data-Driven Insights: Big data analytics provide valuable insights into production performance, enabling continuous improvement and strategic planning.
- Enhanced Supply Chain Integration: Seamless communication and coordination across the supply chain improve transparency, reduce lead times, and enhance overall supply chain resilience.
- Sustainability: Optimized processes and resource management contribute to more sustainable manufacturing practices, reducing environmental impact.
Challenges of Implementing Industry 4.0 Technologies:
- High Initial Investment: The cost of acquiring and integrating advanced technologies can be substantial, posing financial barriers, especially for small and medium-sized enterprises (SMEs).
- Cybersecurity Risks: Increased connectivity and data exchange heighten the vulnerability of manufacturing systems to cyber-attacks and data breaches.
- Skill Gaps: The adoption of Industry 4.0 requires a workforce with specialized skills in digital technologies, data analytics, and system integration, which may be scarce.
- Integration Complexity: Integrating new digital technologies with existing legacy systems can be technically challenging and may disrupt ongoing operations.
- Data Management: Handling large volumes of data, ensuring data quality, and effectively analyzing data to derive actionable insights can be overwhelming.
- Change Management: Organizational resistance to change, lack of leadership commitment, and inadequate training can hinder the successful implementation of Industry 4.0 initiatives.
Overcoming the Challenges:
- Strategic Planning and Investment: Develop a clear roadmap for Industry 4.0 adoption, prioritizing technologies that offer the most significant benefits and aligning investments with long-term business goals.
- Enhancing Cybersecurity: Implement robust cybersecurity measures, including encryption, access controls, and regular security audits, to protect against cyber threats.
- Workforce Development: Invest in training and upskilling programs to equip employees with the necessary digital skills and foster a culture of continuous learning.
- Phased Implementation: Adopt a step-by-step approach to integrating Industry 4.0 technologies, starting with pilot projects to test and refine processes before full-scale deployment.
- Collaborative Partnerships: Partner with technology providers, research institutions, and other organizations to access expertise, share resources, and accelerate technology adoption.
- Effective Data Management: Utilize advanced data management systems and analytics tools to handle large datasets efficiently and extract meaningful insights.
- Leadership and Change Management: Secure strong leadership commitment, communicate the benefits of Industry 4.0 clearly to all stakeholders, and involve employees in the transformation process to reduce resistance and ensure buy-in.
Conclusion: Implementing Industry 4.0 technologies in manufacturing offers substantial benefits in terms of efficiency, flexibility, quality, and sustainability. However, organizations must address challenges related to investment costs, cybersecurity, skill gaps, integration complexity, data management, and change management to realize these benefits fully. By adopting strategic approaches, investing in workforce development, enhancing cybersecurity, and fostering a culture of innovation and collaboration, organizations can overcome these challenges and successfully integrate Industry 4.0 technologies into their manufacturing operations, driving competitive advantage and long-term growth.
8. How does sustainable manufacturing differ from traditional manufacturing, and what practices are essential for achieving sustainability in industrial operations?
Answer:
Sustainable manufacturing emphasizes minimizing environmental impact, conserving resources, and ensuring social responsibility throughout the production process, whereas traditional manufacturing primarily focuses on efficiency and cost-effectiveness without necessarily considering long-term environmental and social implications.
Concepts:
- Sustainable Manufacturing: An approach that integrates environmental, economic, and social considerations into manufacturing processes to create products in a manner that is environmentally responsible, economically viable, and socially equitable.
- Traditional Manufacturing: Focuses on maximizing production output and reducing costs, often prioritizing short-term gains over long-term sustainability.
Differences Between Sustainable and Traditional Manufacturing:
- Resource Efficiency: Sustainable manufacturing prioritizes the efficient use of materials, energy, and water, reducing waste and minimizing resource consumption. Traditional manufacturing may not prioritize resource conservation to the same extent.
- Environmental Impact: Sustainable practices aim to reduce emissions, pollution, and environmental degradation by implementing cleaner production methods and waste management strategies. Traditional manufacturing may result in higher environmental footprints due to less stringent environmental controls.
- Lifecycle Thinking: Sustainable manufacturing considers the entire lifecycle of a product, from design and production to disposal and recycling, ensuring sustainability at each stage. Traditional manufacturing often focuses on the production phase without extensive consideration of the product’s end-of-life impact.
- Social Responsibility: Sustainable manufacturing incorporates fair labor practices, community engagement, and ethical sourcing of materials, ensuring positive social outcomes. Traditional manufacturing may not adequately address social dimensions, potentially leading to labor issues and community conflicts.
- Innovation and Adaptability: Sustainable manufacturing encourages innovation in materials, processes, and technologies to enhance sustainability, whereas traditional manufacturing may resist changes that could disrupt established production efficiencies.
Essential Practices for Achieving Sustainability in Industrial Operations:
- Energy Efficiency: Implement energy-efficient technologies and practices, such as upgrading to energy-efficient machinery, optimizing production processes, and utilizing renewable energy sources.
- Waste Reduction and Recycling: Adopt waste minimization strategies, recycle materials, and implement circular economy principles to reduce the amount of waste generated and promote the reuse of resources.
- Sustainable Material Sourcing: Use eco-friendly and sustainably sourced materials, and prioritize the use of recycled or renewable resources to decrease the environmental impact of raw material extraction.
- Pollution Prevention: Implement measures to control and reduce emissions, effluents, and other pollutants through cleaner production techniques, advanced filtration systems, and proper waste treatment.
- Water Conservation: Optimize water usage in manufacturing processes, implement water recycling systems, and reduce water waste to conserve this vital resource.
- Lifecycle Assessment (LCA): Conduct LCAs to evaluate the environmental impact of products throughout their lifecycle, identifying areas for improvement and making informed decisions to enhance sustainability.
- Green Design: Incorporate eco-design principles that prioritize energy efficiency, material conservation, and ease of recycling during the product design phase.
- Employee Engagement and Training: Foster a culture of sustainability by educating and involving employees in sustainable practices, encouraging innovative ideas, and promoting environmental stewardship.
- Supply Chain Collaboration: Work with suppliers to ensure that sustainable practices are adopted throughout the supply chain, from raw material extraction to final product delivery.
- Compliance and Certification: Adhere to environmental regulations and pursue sustainability certifications, such as ISO 14001, to demonstrate commitment to sustainable manufacturing practices.
Conclusion: Sustainable manufacturing represents a transformative shift from traditional manufacturing by integrating environmental stewardship, resource efficiency, and social responsibility into industrial operations. By adopting essential sustainable practices, manufacturers can reduce their environmental footprint, enhance economic viability, and contribute positively to society. This holistic approach not only ensures long-term sustainability but also positions organizations as leaders in responsible and innovative manufacturing.
9. What are the primary considerations in designing a lean manufacturing system, and how do these considerations contribute to overall operational excellence?
Answer:
Designing a lean manufacturing system involves implementing strategies and practices that eliminate waste, optimize processes, and enhance value creation, thereby contributing to overall operational excellence.
Concepts:
- Lean Manufacturing: A production philosophy focused on minimizing waste and maximizing value through continuous improvement and efficient process management.
- Operational Excellence: Achieving superior performance in manufacturing operations by optimizing processes, enhancing quality, and fostering a culture of excellence.
Primary Considerations in Designing a Lean Manufacturing System:
Value Stream Mapping:
- Definition: A visual tool that outlines all the steps in the production process, identifying value-added and non-value-added activities.
- Contribution: Helps in identifying areas of waste and opportunities for process improvement, ensuring that every step contributes to customer value.
Just-In-Time (JIT) Production:
- Definition: Producing and delivering products only as they are needed in the production process, reducing inventory levels.
- Contribution: Minimizes inventory holding costs, reduces waste from overproduction, and enhances responsiveness to customer demand.
Continuous Improvement (Kaizen):
- Definition: An ongoing effort to improve products, services, and processes incrementally.
- Contribution: Fosters a culture of proactive problem-solving and innovation, leading to sustained operational enhancements and increased efficiency.
Standardized Work:
- Definition: Establishing consistent procedures and practices for performing tasks.
- Contribution: Ensures uniformity in operations, reduces variability, and simplifies training, leading to higher quality and reliability in production.
Waste Elimination (Muda):
- Definition: Identifying and removing non-value-added activities from the production process.
- Types of Waste: Overproduction, waiting, transportation, overprocessing, inventory, motion, and defects.
- Contribution: Streamlines operations, reduces costs, and improves overall efficiency by eliminating activities that do not add value.
Value-Added vs. Non-Value-Added Activities:
- Definition: Differentiating between activities that directly contribute to customer value and those that do not.
- Contribution: Prioritizes resource allocation towards value-added activities, enhancing product quality and customer satisfaction.
Pull Systems (Kanban):
- Definition: A production system where downstream processes signal the need for materials or products, initiating production accordingly.
- Contribution: Aligns production with actual demand, reducing excess inventory and ensuring timely delivery of products.
Flexible Workforce:
- Definition: Training employees to perform multiple tasks and adapt to changing production needs.
- Contribution: Increases workforce adaptability, reduces downtime, and enhances the ability to respond to fluctuations in demand.
5S Methodology:
- Definition: A workplace organization method comprising Sort, Set in order, Shine, Standardize, and Sustain.
- Contribution: Creates a clean, organized, and efficient work environment, improving productivity and reducing the risk of errors and accidents.
Total Quality Management (TQM):
- Definition: An organization-wide approach focused on continuous improvement, customer satisfaction, and quality enhancement.
- Contribution: Integrates quality into every aspect of the manufacturing process, ensuring that products meet or exceed customer expectations.
Contribution to Operational Excellence:
- Enhanced Efficiency: Streamlined processes and waste elimination lead to faster production times and lower operational costs.
- Improved Quality: Standardized work and continuous improvement practices ensure consistent product quality and reduced defect rates.
- Increased Flexibility: A flexible workforce and pull systems allow for quick adaptation to changes in demand and market conditions.
- Higher Employee Engagement: Involving employees in continuous improvement initiatives fosters a sense of ownership and motivation, contributing to a positive work culture.
- Customer Satisfaction: By focusing on value-added activities and quality enhancement, lean manufacturing ensures that customer needs are met effectively, leading to increased satisfaction and loyalty.
- Sustainable Growth: Operational efficiencies and cost savings enable organizations to invest in innovation and expansion, supporting long-term growth and competitiveness.
Conclusion: Designing a lean manufacturing system involves a comprehensive approach that focuses on waste elimination, process optimization, and continuous improvement. By addressing key considerations such as value stream mapping, JIT production, standardized work, and workforce flexibility, organizations can achieve operational excellence, enhancing efficiency, quality, and customer satisfaction. These practices not only improve current performance but also establish a foundation for sustainable and resilient manufacturing operations.
10. How can sustainable manufacturing practices reduce the environmental footprint of industrial operations, and what are some examples of these practices?
Answer:
Sustainable manufacturing practices aim to minimize the environmental footprint of industrial operations by reducing resource consumption, lowering emissions, and promoting the efficient use of materials. These practices contribute to environmental preservation, cost savings, and compliance with regulatory standards.
Concepts:
- Sustainable Manufacturing: The creation of products using processes that minimize negative environmental impacts, conserve energy and natural resources, and are safe for workers and communities.
- Environmental Footprint: The total impact of industrial operations on the environment, including resource use, waste generation, and emissions.
How Sustainable Manufacturing Practices Reduce Environmental Footprint:
Resource Efficiency:
- Energy Conservation: Implementing energy-efficient technologies and optimizing production processes to reduce energy consumption.
- Water Conservation: Using water-saving techniques and recycling water within the manufacturing process to minimize water usage.
Waste Minimization:
- Lean Manufacturing: Reducing waste through process optimization and elimination of non-value-added activities.
- Circular Economy: Designing products for reuse, recycling, and remanufacturing to extend their lifecycle and reduce material waste.
Emission Reduction:
- Pollution Control: Installing advanced filtration and scrubbing systems to capture and reduce air and water pollutants.
- Carbon Footprint Reduction: Adopting renewable energy sources and improving energy efficiency to lower greenhouse gas emissions.
Sustainable Material Sourcing:
- Eco-Friendly Materials: Using renewable, recyclable, or biodegradable materials to reduce environmental impact.
- Responsible Procurement: Selecting suppliers that adhere to sustainable practices and provide environmentally certified materials.
Eco-Design:
- Product Lifecycle Management: Designing products with their entire lifecycle in mind, ensuring ease of disassembly, recycling, and minimal environmental impact.
- Modular Design: Creating products with interchangeable parts to facilitate repairs, upgrades, and recycling.
Green Manufacturing Technologies:
- Additive Manufacturing: Utilizing 3D printing to produce components with less material waste compared to traditional subtractive methods.
- Advanced Manufacturing Processes: Implementing technologies like laser cutting and precision machining to enhance efficiency and reduce waste.
Supply Chain Sustainability:
- Logistics Optimization: Streamlining transportation and distribution to reduce fuel consumption and emissions.
- Supplier Collaboration: Working with suppliers to ensure sustainable practices throughout the supply chain, from raw material extraction to product delivery.
Examples of Sustainable Manufacturing Practices:
- Energy-Efficient Lighting and Machinery: Installing LED lighting and energy-efficient motors to reduce electricity consumption in manufacturing facilities.
- Waste Heat Recovery Systems: Capturing and reusing waste heat from industrial processes to generate additional energy or for heating purposes.
- Solar Power Integration: Incorporating solar panels into manufacturing plants to supply renewable energy and decrease reliance on fossil fuels.
- Closed-Loop Water Systems: Recycling and reusing water within the manufacturing process to minimize freshwater consumption and reduce wastewater discharge.
- Biodegradable Packaging: Using biodegradable or recyclable packaging materials to reduce environmental impact and enhance product sustainability.
- Eco-Friendly Paints and Coatings: Utilizing low-VOC (volatile organic compounds) paints and coatings to decrease air pollution and improve indoor air quality.
- Green Roofs and Vegetation: Implementing green roofs and planting vegetation around manufacturing facilities to absorb rainwater, provide insulation, and enhance biodiversity.
Conclusion: Sustainable manufacturing practices are essential for reducing the environmental footprint of industrial operations. By focusing on resource efficiency, waste minimization, emission reduction, sustainable material sourcing, and eco-design, manufacturers can significantly lower their impact on the environment. Implementing these practices not only contributes to environmental preservation but also offers economic benefits through cost savings, improved efficiency, and enhanced corporate reputation. Embracing sustainability in manufacturing is a strategic imperative that supports long-term business viability and environmental stewardship.
11. What are the critical factors in designing a flexible manufacturing system, and how do these factors enhance production adaptability and efficiency?
Answer:
Designing a flexible manufacturing system (FMS) involves creating a production environment that can adapt quickly and efficiently to changes in product design, production volume, and market demand. Critical factors in designing an FMS include modularity, automation, information systems, workforce versatility, and scalable infrastructure.
Concepts:
- Flexible Manufacturing System (FMS): An integrated system of machines, robots, and computer-controlled tools capable of producing a variety of products with minimal changeover time.
- Production Adaptability: The ability of a manufacturing system to adjust to changes in product types, volumes, and production processes.
- Operational Efficiency: Maximizing productivity and minimizing waste through optimized processes and resource utilization.
Critical Factors in Designing an FMS:
Modularity:
- Definition: Designing production units and equipment that can be easily reconfigured or replaced to accommodate different products.
- Enhancement: Modularity allows for quick adjustments to production lines, enabling manufacturers to switch between products with minimal downtime and cost.
Automation and Robotics:
- Definition: Incorporating automated machinery and robots to perform repetitive, precise, and complex tasks.
- Enhancement: Automation increases production speed, consistency, and accuracy while reducing labor costs and minimizing human error, enhancing overall efficiency.
Advanced Information Systems:
- Definition: Utilizing integrated software systems like Manufacturing Execution Systems (MES) and Enterprise Resource Planning (ERP) to manage production data and operations.
- Enhancement: These systems provide real-time visibility into production processes, enabling better decision-making, coordination, and responsiveness to changes.
Versatile Workforce:
- Definition: Training employees to perform multiple roles and tasks within the manufacturing system.
- Enhancement: A versatile workforce can quickly adapt to different production needs, reducing reliance on specialized labor and enhancing flexibility.
Scalable Infrastructure:
- Definition: Designing manufacturing facilities with the capacity to expand or contract based on production requirements.
- Enhancement: Scalable infrastructure supports growth and allows for adjustments in production volume without significant overhauls, maintaining operational efficiency.
Standardization and Interchangeability:
- Definition: Creating standardized processes and interchangeable parts that can be used across different products.
- Enhancement: Standardization reduces setup times, simplifies maintenance, and ensures consistency in production, facilitating easier adaptation to new products.
Flexible Supply Chain:
- Definition: Developing a supply chain that can respond swiftly to changes in production demands and product variations.
- Enhancement: A flexible supply chain ensures the timely availability of materials and components, supporting the adaptability and efficiency of the manufacturing system.
Quality Control Integration:
- Definition: Embedding quality control measures within the manufacturing process to ensure product consistency and reliability.
- Enhancement: Integrated quality control minimizes defects, reduces rework, and maintains high product standards even when production processes are frequently adjusted.
Enhancements to Production Adaptability and Efficiency:
- Quick Changeovers: Modular and automated systems enable rapid switchovers between different products, reducing downtime and increasing production flexibility.
- Responsive Production: Advanced information systems and a versatile workforce allow for real-time adjustments to production schedules based on market demand and supply chain dynamics.
- Cost Efficiency: Automation and standardization lower operational costs by increasing production speed, reducing waste, and minimizing the need for specialized labor.
- Improved Product Quality: Consistent processes and integrated quality control ensure that product quality remains high, regardless of production changes.
- Scalability: Scalable infrastructure allows manufacturers to adjust production capacity in response to market fluctuations, maintaining efficiency and meeting customer demands effectively.
Conclusion: Designing a flexible manufacturing system involves considering factors such as modularity, automation, advanced information systems, versatile workforce, and scalable infrastructure. These factors collectively enhance production adaptability and efficiency by enabling quick adjustments to changing production needs, optimizing resource utilization, and maintaining high product quality. A well-designed FMS supports operational excellence, reduces costs, and provides a competitive advantage in dynamic and evolving markets.
12. How can predictive maintenance technologies transform maintenance strategies in manufacturing, and what are the benefits of adopting such technologies?
Answer:
Predictive maintenance technologies leverage data analytics, sensors, and machine learning to anticipate equipment failures before they occur, transforming traditional maintenance strategies from reactive and preventive approaches to a more proactive and condition-based model.
Concepts:
- Predictive Maintenance: A maintenance strategy that uses data-driven insights to predict when equipment maintenance should be performed, minimizing downtime and extending asset lifespan.
- Condition-Based Maintenance: Maintenance activities are triggered by real-time monitoring of equipment conditions rather than on a fixed schedule.
Transformation of Maintenance Strategies:
Data Collection and Monitoring:
- Implementation: Installing sensors and IoT devices on machinery to continuously monitor parameters such as temperature, vibration, pressure, and performance metrics.
- Transformation: Provides real-time data on equipment health, enabling continuous assessment and early detection of anomalies.
Data Analysis and Machine Learning:
- Implementation: Utilizing advanced analytics and machine learning algorithms to process collected data, identifying patterns and predicting potential failures.
- Transformation: Transforms raw data into actionable insights, allowing maintenance teams to prioritize and address issues proactively.
Automation and Integration:
- Implementation: Integrating predictive maintenance systems with existing Enterprise Resource Planning (ERP) and Manufacturing Execution Systems (MES) to streamline maintenance workflows.
- Transformation: Automates maintenance scheduling and resource allocation based on predictive insights, enhancing efficiency and coordination.
Decision Support:
- Implementation: Providing maintenance teams with dashboards and alerts that highlight equipment needing attention based on predictive analytics.
- Transformation: Enhances decision-making by prioritizing maintenance activities based on the likelihood and severity of potential failures.
Benefits of Adopting Predictive Maintenance Technologies:
- Reduced Downtime:
- Benefit: By anticipating equipment failures, maintenance can be scheduled during planned downtime, minimizing unexpected interruptions in production.
- Cost Savings:
- Benefit: Predictive maintenance reduces the costs associated with emergency repairs, extends the lifespan of machinery, and lowers the frequency of maintenance activities by addressing issues only when necessary.
- Improved Equipment Performance:
- Benefit: Regular monitoring and timely maintenance ensure that equipment operates at optimal performance levels, enhancing overall production efficiency and product quality.
- Enhanced Safety:
- Benefit: Predictive maintenance helps identify and mitigate potential safety hazards related to equipment malfunctions, protecting workers and reducing the risk of accidents.
- Resource Optimization:
- Benefit: Maintenance resources, including labor and spare parts, are allocated more efficiently based on actual equipment needs, avoiding over-maintenance and reducing inventory costs.
- Data-Driven Insights:
- Benefit: Continuous data collection and analysis provide valuable insights into equipment performance trends, informing future investments in machinery and maintenance practices.
- Sustainability:
- Benefit: Efficient maintenance practices reduce energy consumption and material waste associated with equipment inefficiencies and breakdowns, contributing to more sustainable manufacturing operations.
- Scalability:
- Benefit: Predictive maintenance systems can be scaled across multiple machines and facilities, providing consistent maintenance standards and performance improvements throughout the organization.
Conclusion: Predictive maintenance technologies significantly transform maintenance strategies in manufacturing by enabling a proactive, data-driven approach to equipment management. The adoption of these technologies leads to reduced downtime, cost savings, improved equipment performance, enhanced safety, and optimized resource utilization. By leveraging predictive maintenance, manufacturers can achieve greater operational efficiency, extend the lifespan of their assets, and maintain high standards of product quality and workplace safety, thereby gaining a competitive edge in the industry.
Conclusion: The above questions and detailed answers provide a comprehensive exploration of industrial and manufacturing technologies. Covering essential topics such as additive vs. subtractive manufacturing, automation and robotics, lean manufacturing principles, quality control vs. quality assurance, supply chain management, CAD/CAM integration, Industry 4.0, sustainable manufacturing, flexible manufacturing systems, predictive maintenance, and more, these inquiries encourage students to critically analyze and engage with the complexities of modern manufacturing practices. By delving into these areas, students are equipped with the knowledge and critical thinking skills necessary to excel in the field of industrial and manufacturing technologies, promoting innovation, efficiency, and sustainability in production environments. These questions foster a deeper understanding of how advanced technologies and strategic management practices can be integrated to achieve operational excellence and competitive advantage, making learning an engaging and dynamic process.