Adaptation and Plasticity in Physiology
Adaptation and plasticity are fundamental physiological processes that enable organisms to survive and thrive in dynamic environments. These processes reflect the remarkable ability of living systems to respond to internal and external stimuli—ranging from climate shifts to changes in nutrient availability—by adjusting physiological functions, gene expression patterns, and even developmental pathways. To appreciate this versatility, it is essential to understand how science integrates principles from biology, genetics, and physiology.
Physiological plasticity allows an organism to fine-tune its functions in response to short-term challenges such as temperature changes, while adaptation often involves heritable genetic changes shaped by evolutionary forces. For instance, organisms can modify energy and metabolism to conserve or expend energy under varying environmental pressures. Similarly, processes such as homeostasis help maintain internal equilibrium despite external fluctuations.
The cellular basis of these responses often lies in the mechanisms covered in cell biology, such as cell communication and signal communication. Cells interpret signals from their environment and adjust behavior through complex molecular interactions, such as alterations in gene expression. These changes often affect protein activity, structure, or abundance—crucial for biological flexibility.
At the genetic level, both mutations and regulatory modifications contribute to long-term adaptation. The study of molecular genetics provides key insights into these dynamics, especially when paired with advanced tools such as DNA technology and knowledge of DNA and RNA interactions. These technologies allow researchers to trace adaptive shifts across generations and populations.
The influence of evolutionary biology is evident in how certain traits become widespread through natural selection. This intersection is explored further in molecular evolution, which investigates how genes and proteins evolve in response to environmental challenges. Moreover, the principles of protein synthesis help explain how genetic instructions translate into functional molecules essential for adaptation.
Physiological plasticity is also observed during development. During early stages, cells respond to positional and molecular cues, leading to differentiation and tissue specialization, as discussed in cell development. These outcomes are further governed by the intricacies of the cell cycle and insights from cell structure and cell physiology.
Researchers also consider broader ecological implications. Understanding adaptation requires knowledge of ecology, where interactions with other organisms and environments play a role in shaping phenotypic responses. Furthermore, applied fields such as applications of genetics in medicine harness adaptation principles to personalize treatments and design adaptive therapies.
Ultimately, the study of adaptation and plasticity ties together foundational knowledge from science and genetics with modern discoveries in molecular biology. It emphasizes the dynamic nature of life and the capacity of organisms to adjust, survive, and evolve—offering essential perspectives for aspiring biologists and health scientists alike.
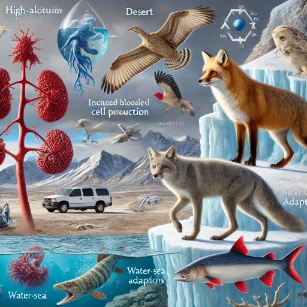
Table of Contents
Short-Term Adaptation: Physiological Plasticity
Short-term physiological plasticity occurs within minutes, hours, or days to maintain homeostasis in response to changing environmental or physiological conditions. Examples include:
Cardiovascular Adjustments During Exercise
When a person engages in physical activity, the cardiovascular system quickly adjusts to meet increased energy demands:
- Increased Heart Rate (HR): The heart pumps faster to deliver oxygen-rich blood to muscles.
- Increased Stroke Volume: The amount of blood pumped per heartbeat increases to improve cardiac output.
- Redistribution of Blood Flow: Blood is diverted away from less active organs (e.g., gastrointestinal tract) toward working muscles.
- Vasodilation: Blood vessels in muscles dilate to enhance blood flow and nutrient delivery.
- Oxygen Utilization: Muscles extract oxygen more efficiently, improving aerobic performance.
This plasticity allows individuals to perform strenuous activities and recover efficiently.
Acclimatization to High Altitude
At high altitudes, oxygen availability in the atmosphere decreases, creating a physiological challenge for humans. Over days to weeks, the body adapts through:
- Increased Breathing Rate (Hyperventilation): To compensate for low oxygen levels, respiratory rates increase.
- Increased Red Blood Cell Production: The hormone erythropoietin (EPO) stimulates the bone marrow to produce more red blood cells, improving oxygen transport.
- Enhanced Hemoglobin Affinity for Oxygen: Hemoglobin molecules optimize their ability to bind and release oxygen.
- Increased Capillary Density: Over time, tissues may develop more capillaries to enhance oxygen delivery to cells.
These changes allow individuals to function effectively in low-oxygen environments, such as mountainous regions.
Thermoregulation in Response to Temperature Changes
- Cold Exposure: The body responds to cold by vasoconstriction (narrowing blood vessels) to conserve heat and by shivering to generate heat through muscle activity.
- Heat Exposure: The body responds to heat through vasodilation (expanding blood vessels) and sweating to release heat via evaporative cooling.
Such mechanisms ensure the body maintains its core temperature within a narrow, optimal range.
Long-Term Adaptation: Evolutionary Changes
Over generations, physiological adaptations occur in response to persistent environmental pressures. These changes are driven by natural selection, where beneficial traits are passed on to future generations:
Adaptations to Hypoxia (Low Oxygen) in Native High-Altitude Populations
- Populations living at high altitudes, such as the Tibetan Plateau or Andes Mountains, have developed unique adaptations:
- Lower hemoglobin concentrations compared to short-term visitors, reducing blood viscosity.
- Increased lung capacity and more efficient oxygen utilization.
- Genetic variations (e.g., EPAS1 gene in Tibetans) that enhance cellular responses to hypoxia.
Adaptations to Extreme Temperatures
- Arctic Animals (Cold Environments): Animals such as polar bears and seals have thick layers of blubber and fur to insulate against cold.
- Desert Animals (Hot Environments): Camels and other desert animals have adaptations like water conservation mechanisms, efficient cooling (e.g., panting), and nocturnal behavior to avoid extreme heat.
Physiological Adaptations in Marine Mammals
Marine mammals like whales and seals have evolved mechanisms to survive underwater during prolonged dives:
- Higher concentrations of myoglobin in muscles to store oxygen.
- Bradycardia (slowing of heart rate) during dives to conserve oxygen.
- Blood flow redistribution to prioritize vital organs (e.g., brain and heart).
Developmental Plasticity
Developmental plasticity refers to long-term physiological changes that occur during early life stages based on environmental stimuli or challenges:
- Nutritional Availability: Poor nutrition during childhood can influence growth patterns, metabolic processes, and disease susceptibility later in life.
- Environmental Factors: Children raised in high-altitude regions develop greater lung capacities compared to those raised at sea level.
This type of plasticity highlights the importance of the environment in shaping physiological outcomes during critical developmental periods.
Cellular and Molecular Adaptations
At the cellular level, organisms exhibit adaptations to stress or changing environments:
- Heat Shock Proteins (HSPs): These proteins are produced in response to heat or stress and help stabilize cellular structures and repair damaged proteins.
- Hormesis: Exposure to low doses of a stressor (e.g., mild oxidative stress or exercise) can induce protective cellular adaptations that improve resilience.
- Epigenetic Changes: Environmental stimuli can modify gene expression without altering the DNA sequence, leading to long-term adaptive changes.
Clinical and Biomedical Relevance of Physiological Plasticity
Understanding adaptation and plasticity has practical applications in medicine and health:
- Exercise Physiology: Regular exercise induces beneficial adaptations in cardiovascular, muscular, and respiratory systems, improving fitness and reducing disease risk.
- Rehabilitation: Physiological plasticity is leveraged in physical therapy to restore function after injury.
- Space Physiology: Astronauts undergo physiological changes (e.g., muscle and bone loss) during microgravity exposure, requiring countermeasures such as resistance training.
- Hypoxia-Induced Disorders: Research on high-altitude adaptations informs treatments for diseases like chronic obstructive pulmonary disease (COPD).
Why Study Adaptation and Plasticity
Understanding Biological Flexibility
Adaptation and plasticity explain how organisms adjust to changing environments. These processes enable survival through structural, behavioral, or physiological changes. Students learn how species cope with stress, climate change, and resource limitations. This knowledge is vital for understanding biodiversity and resilience.
Applications in Evolutionary Biology
Studying adaptation provides insight into how traits evolve over time. Plastic responses can precede genetic adaptations in populations. This highlights the dynamic relationship between environment and evolution. It strengthens students’ understanding of natural selection and species survival.
Relevance to Human Health
Humans exhibit plasticity in response to altitude, diet, and exercise. Understanding these responses informs medicine, nutrition, and sports science. Students explore how individual variability affects health outcomes. It connects biological flexibility to personal and public health.
Implications for Conservation and Ecology
Plasticity helps species survive in rapidly changing ecosystems. Students study how organisms adjust to habitat loss, pollution, and invasive species. This knowledge supports conservation strategies and environmental planning. It prepares students to address ecological challenges with adaptive thinking.
Foundation for Research and Innovation
Adaptation and plasticity provide a framework for studying gene-environment interactions. Students develop hypotheses about flexible biological systems. This understanding is key to innovation in agriculture, climate resilience, and synthetic biology. It fosters creativity in solving complex biological problems.
Conclusion on Adaptation and Plasticity
Adaptation and plasticity are essential for survival and optimal function across diverse environments and challenges. Whether it is the cardiovascular adjustments during exercise, the body’s acclimatization to high altitudes, or evolutionary changes in populations, the ability to respond and adjust demonstrates the dynamic nature of physiological processes. By studying these mechanisms, we gain valuable insights into health, disease, and the resilience of living organisms.Adaptation and Plasticity: Review Questions with Answers:
Question 1: Define phenotypic plasticity and provide an example.
Answer: Phenotypic plasticity refers to the ability of an organism with a given genotype to change its phenotype in response to changes in the environment. An example is the water flea (Daphnia magna), which can develop protective spines when exposed to chemical cues from predators.
Question 2: How does phenotypic plasticity differ from genetic adaptation?
Answer: Phenotypic plasticity involves reversible changes within an individual’s lifetime in response to environmental conditions, without altering the genetic code. In contrast, genetic adaptation involves changes in the genetic makeup of a population over generations through natural selection, leading to inherited traits that enhance survival and reproduction.
Question 3: What is developmental plasticity, and how does it manifest in organisms?
Answer: Developmental plasticity is a form of phenotypic plasticity where an organism’s development can be altered by environmental conditions, leading to permanent changes in morphology, physiology, or behavior. For instance, in some reptiles, the temperature at which eggs are incubated determines the sex of the offspring, a phenomenon known as temperature-dependent sex determination.
Question 4: Explain the concept of reaction norms in the context of phenotypic plasticity.
Answer: A reaction norm is a graphical representation that depicts the range of phenotypes an organism can develop from a single genotype across a variety of environmental conditions. It illustrates how different environments can influence the expression of traits, highlighting the organism’s capacity for phenotypic plasticity.
Question 5: What role does phenotypic plasticity play in an organism’s ability to cope with climate change?
Answer: Phenotypic plasticity allows organisms to adjust their physiology, behavior, or development in response to changing environmental conditions, such as those induced by climate change. This flexibility can enhance survival in the short term by enabling individuals to maintain functionality despite new stresses. For example, some plants can alter their flowering time in response to temperature changes, ensuring reproductive success.
Question 6: Describe an example of phenotypic plasticity in animals in response to predation.
Answer: The red-eyed tree frog (Agalychnis callidryas) exhibits phenotypic plasticity through variable hatching times. When embryos sense vibrations from potential predators, such as snakes, they can hatch early to escape, even though early hatching may expose them to other risks. This adaptive response increases their chances of survival in the presence of immediate threats.
Question 7: How does phenotypic plasticity contribute to evolutionary processes?
Answer: Phenotypic plasticity can influence evolutionary processes by allowing organisms to survive in varying environments, potentially exposing them to new selective pressures. Over time, if certain plastic responses confer a significant survival or reproductive advantage, they may become genetically assimilated into the population, leading to evolutionary changes.
Question 8: What is the difference between acclimatization and adaptation?
Answer: Acclimatization refers to the physiological adjustments an individual organism makes in response to changes in its environment, typically reversible and occurring within its lifetime. Adaptation, on the other hand, involves genetic changes in a population over multiple generations, resulting in traits that are heritable and enhance the population’s fitness in a specific environment.
Adaptation and Plasticity: Thought-Provoking Questions
Based on the topic of adaptation and phenotypic plasticity, here are 12 thought-provoking questions along with detailed and elaborate answers:
Question 1: How does phenotypic plasticity influence the evolutionary trajectory of a species?
Answer: Phenotypic plasticity allows organisms to exhibit different phenotypes in response to varying environmental conditions without genetic changes. This flexibility can influence evolution in several ways:
Immediate Adaptation: Plasticity enables individuals to survive and reproduce in changing environments by adjusting their physiology, behavior, or morphology.
Genetic Accommodation: Over time, plastic responses that confer a fitness advantage can lead to genetic changes that stabilize these traits, a process known as genetic accommodation.
Genetic Assimilation: If a plastic trait becomes advantageous in a consistently encountered environment, natural selection may favor genetic mutations that fix this trait, reducing the reliance on environmental triggers—a process called genetic assimilation.
Thus, phenotypic plasticity can act as a catalyst for evolutionary change by allowing populations to explore a range of phenotypes, some of which may become genetically encoded over time.
Question 2: In what ways can phenotypic plasticity be considered both beneficial and detrimental to an organism?
Answer: Phenotypic plasticity offers several advantages:
Survival in Variable Environments: Organisms can adjust to different environmental conditions, enhancing their chances of survival and reproduction.
Resource Optimization: Plasticity allows for efficient utilization of available resources by modifying physiological or behavioral traits.
However, there are potential drawbacks:
Energy Costs: Maintaining the machinery for plastic responses can be energetically expensive.
Maladaptive Responses: Inaccurate environmental cues can lead to inappropriate phenotypic changes, reducing fitness.
Delayed Adaptation: Reliance on plasticity might delay genetic adaptations that could be more beneficial in the long term.
Therefore, while phenotypic plasticity provides immediate adaptive benefits, it also comes with costs and risks that can influence an organism’s fitness.
Question 3: How does phenotypic plasticity differ between plants and animals, considering their mobility and environmental interactions?
Answer: Phenotypic plasticity manifests differently in plants and animals due to their distinct lifestyles:
Plants: Being sessile, plants rely heavily on plasticity to cope with environmental variability. They can alter their growth patterns, leaf morphology, and root systems in response to factors like light, water, and nutrient availability. For example, plants may develop larger leaves in shaded conditions to capture more light.
Animals: While animals can move to favorable environments, they also exhibit plasticity in behavior, physiology, and morphology. For instance, some fish can adjust their gill surface area to optimize oxygen uptake in varying water conditions.
In summary, both plants and animals utilize phenotypic plasticity, but the extent and nature of their plastic responses are shaped by their mobility and specific environmental interactions.