Nuclear Fission: A Fundamental Nuclear Process
Nuclear fission is a process in which a heavy atomic nucleus splits into two or more lighter nuclei, releasing a significant amount of energy. It is a central topic in nuclear physics and a pivotal concept in the broader discipline of physics. Understanding nuclear fission requires a solid foundation in modern physics, including the subatomic behavior explored in atomic physics and the fundamental particle interactions studied in particle physics.
The mechanism behind fission involves complex interactions among fermions like protons and neutrons, and is governed by the fundamental forces of nature. These interactions are mathematically described by quantum field theory, which provides a unified framework for particle interactions. Fission is not an isolated phenomenon—it must be understood in relation to nuclear fusion, its counterpart in energy production and astrophysics.
The probability and conditions under which a nucleus undergoes fission are influenced by principles of quantum mechanics. Concepts such as quantum tunneling, quantum superposition, and the uncertainty principle play key roles in determining the behavior of nuclear particles. The probabilistic interpretation of outcomes is captured in the wave function, while wave-particle duality further explains the dual characteristics of particles within the nucleus.
Fission processes are governed by energy balances described by statistical mechanics, which also helps model the distribution of released energy and emitted particles. This energy release is not merely theoretical; it underpins technologies such as nuclear reactors and weapons, which are built upon controlled or uncontrolled nuclear reactions. The fission process can produce radioactive isotopes, adding further complexity and relevance to fields like medical imaging and nuclear waste management.
To fully grasp the implications of fission, students must also consider the broader context of relativity, especially mass-energy equivalence, and the interactions of subatomic particles such as bosons responsible for force mediation. Furthermore, understanding how fission fits into quantum entanglement phenomena can offer insights into the interconnected nature of particles post-reaction. Related studies in condensed matter physics explore how nuclear properties influence material behavior in various states of matter.
To build a foundation for nuclear fission, one must first become familiar with the structure of the atom and delve into the rules of quantum numbers and electron configuration. These topics, explored in detail through atomic physics, serve as the conceptual gateway to understanding the mechanisms and impact of nuclear fission, a phenomenon that continues to shape both scientific progress and societal development.
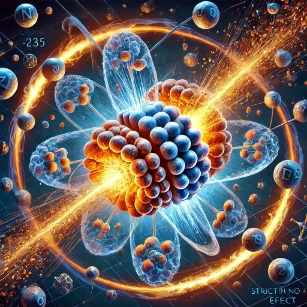
Typical Reaction:
One of the most common fission reactions involves uranium-235. When a neutron collides with a uranium-235 nucleus, it becomes unstable and splits into smaller nuclei. A typical reaction can be expressed as:- Reactants: A uranium-235 nucleus and a neutron.
- Products: Krypton-92, barium-141, three free neutrons, and a significant amount of energy.
- The specific isotopes of krypton and barium may vary slightly due to the probabilistic nature of the process.
Chain Reaction:
The fission process has a self-sustaining potential due to the release of neutrons:- The free neutrons released in the initial reaction may collide with other uranium-235 nuclei, causing them to undergo fission.
- This secondary fission generates additional neutrons, which can induce further fission reactions.
- The process can exponentially amplify, forming what is called a chain reaction.
- Controlled Chain Reaction: In nuclear power plants, the chain reaction is carefully moderated using control rods that absorb excess neutrons, maintaining a steady and safe energy output.
- Uncontrolled Chain Reaction: In nuclear weapons, the chain reaction occurs explosively, releasing energy in a fraction of a second.
Energy Release:
The energy from nuclear fission is predominantly released in the form of:- Kinetic Energy: The fission fragments (e.g., krypton and barium) move at extremely high speeds.
- Thermal Energy: This energy heats surrounding materials.
- Radiation: High-energy photons (gamma rays) and other forms of radiation are emitted.
- Neutron Energy: Free neutrons carry away kinetic energy.
Applications:
- Nuclear Power Generation:
- Controlled fission reactions are utilized in nuclear reactors to generate electricity.
- Reactors use fuels like uranium-235 or plutonium-239.
- Heat from fission is used to convert water into steam, which drives turbines to produce electricity.
- Reactor types include Pressurized Water Reactors (PWRs), Boiling Water Reactors (BWRs), and Fast Breeder Reactors (FBRs).
- Nuclear Weapons:
- Uncontrolled chain reactions are harnessed in nuclear bombs for destructive purposes.
- Examples include the bombs dropped on Hiroshima and Nagasaki during World War II, which relied on uranium-235 and plutonium-239.
- Medical Applications:
- Nuclear reactors produce isotopes like technetium-99m, used in medical imaging.
- Neutron sources from fission are used in cancer treatments and sterilization of medical equipment.
- Scientific Research:
- Fission provides insights into nuclear physics and enables experiments with neutron scattering.
- Research reactors support advancements in material science, biology, and chemistry.
- Space Exploration:
- Fission reactors or radioisotope thermoelectric generators (RTGs) are used to power spacecraft, such as the Voyager and Mars rovers.
Environmental and Safety Considerations:
- Advantages:
- High energy density: A small amount of fuel generates vast amounts of energy.
- Reduced greenhouse gas emissions compared to fossil fuels.
- Long-term energy supply from advanced reactor designs and breeder reactors.
- Challenges:
- Nuclear waste: Fission produces radioactive waste that requires secure, long-term storage.
- Risk of accidents: Reactor malfunctions, such as in Chernobyl or Fukushima, can have catastrophic consequences.
- Proliferation concerns: The potential for misuse of fission materials in weapons.
Why Study Nuclear Fission
Mechanism of Energy Release in Nuclear Reactors
Nuclear fission involves splitting a heavy nucleus into smaller nuclei, releasing a large amount of energy. Students study how chain reactions are initiated and controlled in nuclear reactors. Understanding the fission process is essential for analyzing reactor design and efficiency. It provides insight into one of the primary methods of nuclear energy production.
Applications in Energy and Industry
Fission is the backbone of nuclear power plants, which supply electricity to millions. Students explore how uranium and plutonium are used as fuel, and how reactors convert nuclear energy into heat and electricity. This knowledge supports efforts to transition to low-carbon energy sources. It also informs nuclear fuel cycle management and waste disposal strategies.
Reactor Safety and Risk Management
Students examine how control rods, coolant systems, and containment structures maintain safe operation. They analyze historical incidents to understand lessons in reactor safety. This knowledge builds responsibility and awareness in nuclear engineering. It emphasizes the importance of ethical and technical safeguards.
Nuclear Waste and Environmental Considerations
Fission generates radioactive waste that must be safely stored and managed. Students study the long-term impacts of spent fuel, reprocessing options, and storage technologies. This prepares them to address environmental and sustainability challenges. It promotes responsible stewardship of nuclear materials.
Role in Weapons and International Policy
Fission underlies the technology of nuclear weapons, raising important ethical and security considerations. Students explore arms control treaties, proliferation risks, and geopolitical dynamics. This understanding informs policy debates and global security efforts. It equips students to engage critically with the societal implications of nuclear technology.
Summary
In summary, nuclear fission is a powerful process with transformative applications in energy, medicine, and science. However, it also demands meticulous management to mitigate risks and maximize its benefits. In summary, nuclear fission is a highly potent process that has revolutionized various sectors, particularly in energy production, medicine, and scientific research. In the energy sector, controlled nuclear fission reactions serve as the backbone of nuclear power plants, providing a substantial and consistent supply of electricity with relatively low greenhouse gas emissions compared to fossil fuels. In medicine, fission reactors enable the production of vital radioactive isotopes used in diagnostics and cancer treatments, significantly advancing healthcare capabilities. Additionally, scientific research benefits from nuclear fission through the generation of neutron sources for material analysis and experimental physics, contributing to technological and scientific progress.Review Questions and Answers:
What is nuclear fission and what happens during this process?
Answer: Nuclear fission is the splitting of a heavy atomic nucleus into two or more lighter nuclei, accompanied by the release of energy and neutrons. This process results from the instability of the nucleus and is the basis for both nuclear reactors and weapons.How do neutrons contribute to sustaining a fission chain reaction?
Answer: Neutrons released during fission can collide with other fissile nuclei, triggering further fission events. If enough neutrons are produced and not absorbed by non-fissile material, a self-sustaining chain reaction occurs.What is meant by the term “critical mass” in nuclear fission?
Answer: Critical mass is the minimum amount of fissile material required to maintain a self-sustaining chain reaction. Below this mass, too many neutrons escape, and the reaction dies out; above it, the reaction can escalate uncontrollably.How is energy released during nuclear fission explained by mass defect and Einstein’s equation?
Answer: The mass of the resulting fission fragments is less than that of the original nucleus; the missing mass (mass defect) is converted into energy according to Einstein’s equation E = mc², releasing significant energy during fission.What factors affect the rate of a fission chain reaction in a nuclear reactor?
Answer: The rate is influenced by the concentration and purity of fissile material, the geometry and arrangement of the reactor core, the presence of neutron moderators and reflectors, and control mechanisms such as control rods.How do control rods function in a nuclear fission reactor?
Answer: Control rods, made of materials that absorb neutrons, are inserted into or withdrawn from the reactor core to regulate the number of free neutrons, thereby controlling the rate of the fission chain reaction and ensuring safe operation.What distinguishes induced fission from spontaneous fission?
Answer: Induced fission occurs when an external neutron is absorbed by a nucleus, triggering its splitting, while spontaneous fission happens without any external trigger, though it is much less common in fissile materials.How is the energy from nuclear fission harnessed to generate electricity?
Answer: In nuclear reactors, the energy released as heat from fission is used to produce steam, which drives turbines connected to generators, converting thermal energy into electrical power.What are the potential applications and implications of nuclear fission in modern technology?
Answer: Nuclear fission is used in power generation, medical isotope production, and scientific research. It also underpins the design of nuclear weapons, raising important safety, security, and environmental concerns.How does the concept of neutron economy influence reactor design and safety?
Answer: Neutron economy refers to the balance between neutron production and absorption. Efficient reactor design maximizes useful fission reactions while minimizing neutron losses, ensuring a stable and controllable chain reaction that is crucial for both energy production and safety.
Thought-Provoking Questions and Answers:
How might advances in nuclear fission technology contribute to a more sustainable energy future?
Answer: Advances such as improved fuel utilization, enhanced reactor safety, and the development of small modular reactors could make nuclear fission a cleaner and more flexible energy source. Innovations may reduce waste and increase efficiency, helping to lower greenhouse gas emissions in a low-carbon future.What are the key challenges in achieving a controlled and sustained fission reaction for power generation?
Answer: The challenges include maintaining an optimal neutron balance, ensuring precise control over the reaction rate with control rods and moderators, managing heat removal from the reactor core, and preventing accidental criticality. Addressing these issues is essential for safe and efficient reactor operation.How could next-generation reactor designs, such as fast reactors or molten salt reactors, change the landscape of nuclear energy?
Answer: Next-generation reactors offer potential improvements in safety, fuel efficiency, and waste reduction. Fast reactors can utilize fuel more completely, while molten salt reactors operate at lower pressures and allow for online fuel processing, possibly revolutionizing how nuclear energy is generated and managed.What impact do you think nuclear fission research will have on non-energy applications, such as medicine or space exploration?
Answer: Nuclear fission research can lead to advances in medical isotope production for diagnostics and treatment, and in space exploration through compact nuclear power systems that provide reliable energy for long-duration missions. These applications could drive breakthroughs in healthcare and enable deeper space exploration.How does the concept of critical mass influence the design and safety protocols of nuclear facilities?
Answer: Critical mass determines the minimum amount of fissile material needed for a chain reaction. Facilities must be designed to avoid accidental assembly of a supercritical mass by controlling material geometry and separation, which informs safety protocols, storage practices, and emergency response strategies.What role do international regulations and treaties play in balancing the peaceful use of nuclear fission with non-proliferation goals?
Answer: International regulations and treaties, such as the Non-Proliferation Treaty (NPT), set standards and guidelines for the safe and secure use of nuclear technology. They help prevent the spread of nuclear weapons by monitoring and controlling fissile material while promoting cooperation for peaceful energy applications.How can improvements in computational modeling and simulation enhance our understanding of fission processes and reactor behavior?
Answer: Advanced modeling enables detailed simulations of nuclear reactions, neutron transport, and thermal-hydraulic behavior in reactors. These tools help optimize reactor design, predict potential failure modes, and develop innovative safety systems, ultimately leading to more reliable and efficient nuclear power plants.In what ways might public perception of nuclear fission change if breakthrough technologies significantly improve reactor safety and waste management?
Answer: If breakthrough technologies lead to reactors that are demonstrably safer and produce less long-lived waste, public perception could shift toward greater acceptance of nuclear power. Increased transparency, improved risk communication, and successful demonstration projects would be key in building trust with the public.What ethical considerations should be taken into account when developing and deploying nuclear fission technologies, especially in the context of nuclear weapons?
Answer: Ethical considerations include the potential for mass destruction, long-term environmental contamination, and the risk of nuclear proliferation. Developers and policymakers must balance the benefits of nuclear technology for energy and medicine with the moral responsibility to prevent misuse and ensure global security.How might emerging trends in renewable energy and energy storage technologies influence the future role of nuclear fission in the global energy mix?
Answer: As renewable energy and storage technologies advance, nuclear fission may serve as a stable, baseload power source that complements intermittent renewables. Its role could evolve to support grid stability and reduce carbon emissions, provided that safety, waste, and cost challenges are effectively addressed.What innovations in fuel cycle management could reduce nuclear waste and improve the sustainability of fission-based power generation?
Answer: Innovations such as reprocessing spent fuel, developing advanced fuel types with higher burnup rates, and employing closed fuel cycles can reduce waste and recycle valuable materials. These improvements can enhance the sustainability and economic viability of nuclear energy.How do you envision the integration of nuclear fission technologies with emerging digital control systems and artificial intelligence?
Answer: Integrating digital control systems and AI could lead to smarter reactors capable of real-time monitoring, predictive maintenance, and autonomous safety responses. These systems may optimize reactor performance, enhance operational safety, and reduce human error, paving the way for the next generation of nuclear power plants.
Numerical Problems and Solutions:
Calculate the energy released in MeV when a single fission event of uranium-235 produces a mass defect of 0.2 u. (1 u = 931.5 MeV)
Solution: Energy = 0.2 u × 931.5 MeV/u = 186.3 MeV.A reactor operates at a power level of 1000 MW. If each fission event releases 200 MeV, determine the number of fissions per second.
Solution:
• Convert 200 MeV to joules: 200 MeV = 200 × 1.602×10⁻¹³ J = 3.204×10⁻¹¹ J.
• Power in joules per second: 1000 MW = 1.0×10⁹ W.
• Fissions per second = 1.0×10⁹ J/s / 3.204×10⁻¹¹ J ≈ 3.12×10¹⁹ fissions/s.Determine the decay constant (λ) for an isotope with a half-life of 30 years. (1 year = 3.156×10⁷ s)
Solution:
• t₁/₂ = 30 × 3.156×10⁷ s = 9.468×10⁸ s.
• λ = ln(2) / t₁/₂ = 0.693 / 9.468×10⁸ s ≈ 7.32×10⁻¹⁰ s⁻¹.A sample initially contains 1.0×10²⁰ radioactive atoms. How many atoms remain after 10 half-lives?
Solution:
Remaining atoms = 1.0×10²⁰ × (1/2)¹⁰ = 1.0×10²⁰ / 1024 ≈ 9.77×10¹⁶ atoms.If a control rod reduces the neutron flux by 5.0×10¹² neutrons/m²·s and the fission cross-section is 1.0×10⁻²⁸ m², what is the reduction in the fission rate per square meter?
Solution:
Reduction = neutron flux × cross-section = 5.0×10¹² × 1.0×10⁻²⁸ = 5.0×10⁻¹⁶ fissions/m²·s.A nuclear explosion releases energy equivalent to 20 kilotons of TNT. (1 kiloton TNT = 4.184×10¹² J) Calculate the total energy in joules.
Solution:
Total energy = 20 × 4.184×10¹² J = 8.368×10¹³ J.A reactor contains 1000 kg of U-235. Calculate the total energy released if all the material undergoes fission, assuming each fission releases 200 MeV.
Solution:
• Molar mass of U-235 = 235 g/mol.
• Number of moles = 1.0×10⁶ g / 235 g/mol ≈ 4255 mol.
• Number of nuclei = 4255 mol × 6.022×10²³ nuclei/mol ≈ 2.56×10²⁷ nuclei.
• Energy per fission = 200 MeV = 200 × 1.602×10⁻¹³ J = 3.204×10⁻¹¹ J.
• Total energy = 2.56×10²⁷ × 3.204×10⁻¹¹ J ≈ 8.20×10¹⁶ J.In a fission chain reaction, if each fission produces on average 2.5 neutrons and a reactor starts with 1.0×10¹² neutrons, how many neutrons are present after 5 generations (assuming no losses)?
Solution:
Neutrons after 5 generations = 1.0×10¹² × (2.5)⁵ = 1.0×10¹² × 97.65625 ≈ 9.77×10¹³ neutrons.Calculate the Q-value in joules for a fission reaction that releases 190 MeV of energy.
Solution:
Q-value = 190 MeV = 190 × 1.602×10⁻¹³ J ≈ 3.0438×10⁻¹¹ J.The fission cross-section of U-235 at thermal energies is 580 barns. Express this value in square meters.
Solution:
1 barn = 1.0×10⁻²⁸ m², so 580 barns = 580 × 1.0×10⁻²⁸ m² = 5.8×10⁻²⁶ m².In a reactor, if the average neutron flux is 1.0×10¹⁴ neutrons/m²·s and the fission cross-section is 5.8×10⁻²⁶ m², calculate the fission rate per nucleus per second.
Solution:
Fission rate = neutron flux × cross-section = 1.0×10¹⁴ × 5.8×10⁻²⁶ = 5.8×10⁻¹² s⁻¹.A radioactive sample has an activity of 2.0×10⁶ Bq. How many decays occur in one hour?
Solution:
Decays per hour = 2.0×10⁶ Bq × 3600 s = 7.2×10⁹ decays.