Interaction Between Black Holes and Dark Matter
The interaction between black holes and dark matter is a frontier in modern physics that bridges the known and the unknown. While astrophysics has provided detailed observational evidence of black holes and the gravitational effects of dark matter, how these two entities influence one another remains a deeply theoretical yet promising research area. Insights into this interaction could yield breakthroughs in understanding galactic dynamics, the nature of dark matter particles, and even the fate of the cosmos.
Observations suggest that dense concentrations of dark matter may surround black holes or be pulled into them. This leads scientists to hypothesize that dark matter contributes to the growth and evolution of black holes over cosmic timescales. This idea ties closely to broader questions in cosmology, particularly the Big Bang Theory and the structure and evolution of the universe. Dark matter’s role in seeding galactic centers, which later become sites of supermassive black holes, remains under investigation.
Several models posit that black holes may act as natural laboratories for studying dark matter interactions, especially near event horizons. Theoretical frameworks also explore how Hawking radiation might be influenced or altered by surrounding dark matter density. If such effects are measurable, they could provide indirect detection strategies for dark matter that complement searches in particle accelerators and underground detectors.
The relationship may also extend into stellar evolution, as massive stars that collapse into black holes interact with dark matter differently during their life cycles and remnant phases. For instance, exotic dark matter particles could affect the core-collapse mechanism or influence outcomes like neutron stars and white dwarfs. These stellar remnants might further accrete dark matter, subtly changing their thermodynamic and rotational characteristics.
On a galactic scale, the role of celestial mechanics is key to tracking the gravitational footprints of dark matter near black holes. Studies rooted in classical mechanics and analytical mechanics are increasingly integrated with relativistic corrections and computational models. These efforts are further informed by data from stellar spectroscopy and the study of variable stars, helping to identify gravitational anomalies that could signify dark matter concentrations or hidden black holes.
Researchers also explore how continuum mechanics might describe exotic states of matter influenced by intense gravity and unseen particles. Hypothetical phenomena such as boson stars, primordial black holes, or self-interacting dark matter could change the morphology of galaxies or impact dark energy dynamics on large scales.
Advancing these theories depends on the synthesis of knowledge from stellar physics, including processes such as nuclear fusion and stellar nucleosynthesis. They shape the environments where dark matter may accumulate or influence gravitational collapse. At the cosmological end, discussions of the multiverse and its inflationary epochs challenge our understanding of black holes and dark matter as isolated systems, suggesting instead an interconnected fabric that spans multiple possible universes.
As the evidence grows, the intersection between black holes and dark matter offers one of the most promising paths toward unveiling the universe’s hidden architecture. It compels scientists and students alike to reevaluate familiar concepts and delve into unexplored dimensions of matter, energy, and space-time.
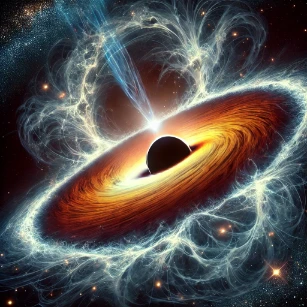
Table of Contents
Supermassive Black Holes and Dark Matter in Galactic Centers
Galactic Centers and Dark Matter Distribution
At the centers of most large galaxies, including the Milky Way, reside supermassive black holes (SMBHs) with masses ranging from millions to billions of solar masses. These black holes significantly influence their surrounding environment, and their interaction with dark matter could shape the structure of galaxies.Dark Matter Density Profiles in Galactic Cores
- Standard Dark Matter Halos: According to Cold Dark Matter (CDM) models, dark matter forms halo-like structures around galaxies. These halos are thought to have a cuspy density profile, where the dark matter density increases sharply toward the center.
- Influence of Supermassive Black Holes: The immense gravitational pull of SMBHs may cause a dark matter spike, where dark matter particles are drawn inward, dramatically increasing the dark matter density near the black hole. This concentration can impact both the evolution of the black hole and the galactic core.
Adiabatic Contraction
- Process: As baryonic matter (gas, dust, and stars) collapses into the galactic center to form stars and the SMBH, it deepens the gravitational potential well, pulling dark matter closer.
- Effect: This effect could lead to a denser dark matter concentration near the SMBH, potentially affecting star formation and galactic dynamics.
Feedback Mechanisms
- AGN Feedback: Active Galactic Nuclei (AGN) powered by SMBHs release enormous amounts of energy, potentially expelling dark matter and gas from the galactic center.
- Impact on Dark Matter: This feedback could smooth out dark matter cusps, leading to a more core-like density profile instead of a sharp spike.
Dark Matter Accretion onto Black Holes
Dark Matter Capture by Black Holes
Black holes grow by accreting matter, and dark matter could contribute to this growth through gravitational capture. Although dark matter interacts weakly with baryonic matter, it is still influenced by gravity and can be drawn toward black holes.Mechanisms of Dark Matter Accretion
- Direct Accretion: Dark matter particles passing close to a black hole can be captured if they lose enough energy through gravitational interactions.
- Self-Annihilating Dark Matter: If dark matter consists of particles that can self-annihilate (like certain models of WIMPs), dense regions around black holes could produce detectable signals (gamma rays, neutrinos) due to frequent annihilations.
Impact on Black Hole Growth
- Early Universe: During the early universe, dark matter accretion may have contributed to the rapid growth of black holes, possibly explaining the presence of massive SMBHs in young galaxies.
- Black Hole Mass Increase: Although dark matter does not interact electromagnetically, gravitational interactions can still lead to significant dark matter accretion over cosmic timescales, subtly increasing the mass of black holes.
Detectable Signals from Dark Matter Annihilation
- Gamma-Ray Excess: Observations of the Galactic Center by telescopes like Fermi Gamma-ray Space Telescope have detected an excess of gamma rays, possibly resulting from dark matter annihilation near the SMBH.
- Neutrino Emissions: Dark matter interactions near black holes might also produce high-energy neutrinos, detectable by observatories like IceCube.
Primordial Black Holes as Dark Matter Candidates
Definition and Formation
Primordial Black Holes (PBHs) are hypothetical black holes that could have formed in the early universe, shortly after the Big Bang, due to extreme density fluctuations. Unlike stellar-mass black holes, PBHs could have a wide range of masses, from tiny subatomic sizes to several solar masses.Primordial Black Holes and Dark Matter
- Dark Matter Candidate: Some theories propose that PBHs could account for part or all of the universe’s dark matter. If PBHs exist in large enough numbers, their combined mass could explain the missing mass inferred from gravitational effects.
- Mass Ranges: PBHs could have formed with various masses, but current research focuses on those between 10⁻¹⁶ and 10² solar masses.
Observational Constraints on PBHs
- Microlensing Surveys: Gravitational microlensing surveys like MACHO, EROS, and OGLE have searched for evidence of PBHs by observing how their gravity bends the light of background stars. These surveys have ruled out PBHs as the dominant form of dark matter in some mass ranges.
- Cosmic Microwave Background (CMB): PBHs could distort the CMB by accreting matter and emitting radiation, leaving observable imprints. Measurements by Planck and other CMB experiments place constraints on the abundance of PBHs.
- Gravitational Wave Observations: The detection of gravitational waves by LIGO and VIRGO from merging black holes has led to speculation that some detected black holes could be primordial. However, more evidence is required to confirm this.
Advantages and Challenges of the PBH Hypothesis
- Advantages:
- No need to introduce new particles beyond the Standard Model.
- PBHs naturally interact via gravity, explaining dark matter’s gravitational effects.
- Challenges:
- Observational constraints from microlensing and the CMB limit the allowable abundance of PBHs.
- Lack of direct evidence for their existence.
Theoretical Models and Simulations in Interaction Between Black Holes and Dark Matter
Simulating Black Hole–Dark Matter Interactions
Astrophysicists use cosmological simulations to model the interaction between black holes and dark matter in galaxies. These simulations explore how dark matter influences black hole formation and growth.- Dark Matter Spikes: Simulations suggest that dark matter could form spikes around black holes, increasing dark matter density near SMBHs.
- Feedback Effects: SMBH-driven feedback, like powerful outflows and jets, may alter the distribution of dark matter in galactic centers.
Dark Matter as a Driver for Black Hole Formation
- Seeding Supermassive Black Holes: Dense dark matter regions could act as seeds for the formation of supermassive black holes in the early universe.
- Influence on Structure Formation: Dark matter’s gravitational pull helps funnel gas and stars into the central regions of galaxies, potentially accelerating black hole growth.
Observational Prospects and Future Research on
Interaction Between Black Holes and Dark Matter:
Next-Generation Observatories
- James Webb Space Telescope (JWST): May provide insights into early black hole formation and dark matter behavior in young galaxies.
- LISA (Laser Interferometer Space Antenna): Could detect gravitational waves from black hole mergers across cosmic history, possibly involving PBHs.
- Cherenkov Telescope Array (CTA): Will search for gamma-ray signals from dark matter annihilation near black holes.
Unresolved Questions
- How much does dark matter contribute to black hole growth?
- Can dark matter annihilation signals near black holes be detected?
- Are primordial black holes a viable dark matter candidate?
Why Study Interaction Between Black Holes and Dark Matter
Unveiling Cosmic Dynamics
Black holes and dark matter both shape the structure and evolution of galaxies. Students explore how their interactions influence galaxy dynamics and formation. This research links gravitational collapse with invisible matter. It deepens understanding of cosmic evolution.
Dark Matter Accretion and Halo Structures
Students study how black holes may accrete dark matter and alter its distribution. This affects halo shapes, star formation, and central galactic regions. Analyzing these effects supports advanced modeling in astrophysics. It bridges large-scale structure with compact objects.
Indirect Detection and Observational Clues
Interactions may produce signals such as radiation or particle decay near black holes. Students learn how to search for indirect evidence of dark matter through black hole environments. These studies guide observational campaigns and detector design. They enhance the search for dark matter.
Testing Fundamental Physics
Black hole–dark matter interactions offer a natural laboratory for testing new theories. Students explore how modifications of gravity or particle physics could explain observations. This supports the development of alternative frameworks beyond current models. It contributes to the evolution of fundamental physics.
Opportunities for Discovery and Research
This interdisciplinary area connects astrophysics, cosmology, and particle physics. Students contribute to simulations, telescope missions, and theoretical developments. It provides cutting-edge training for research careers. It holds the potential to answer major scientific questions.
Interaction Between Black Holes and Dark Matter: Conclusion
The interaction between black holes and dark matter represents a critical frontier in astrophysics. From influencing the structure of galaxies to potentially explaining the elusive nature of dark matter, black holes may hold the key to answering some of the most profound cosmic questions. Theoretical models suggest that dark matter may shape black hole growth, while primordial black holes could be a significant component of dark matter itself. Ongoing and future observations will continue to explore this deep connection, potentially leading to groundbreaking discoveries about the hidden universe.Interaction Between Black Holes and Dark Matter: Review Questions and Answers
What is dark matter and what role does it play in cosmic structures?
Answer: Dark matter is an invisible form of matter that does not interact with light but exerts gravitational influence. It provides the gravitational scaffolding for galaxies and clusters, guiding their formation and influencing their rotational dynamics.How do black holes interact with dark matter in galactic centers?
Answer: Black holes interact with dark matter by exerting intense gravitational forces that can attract and accumulate dark matter particles. This interaction can affect the density profiles around galactic centers and influence the growth and dynamics of both the black hole and its host galaxy.What observational evidence supports the idea that dark matter surrounds black holes?
Answer: Observational evidence includes the analysis of galaxy rotation curves, gravitational lensing effects, and the study of stellar orbits near galactic centers. These data indicate mass distributions that require the presence of unseen matter, consistent with dark matter halos interacting with central black holes.How do computer simulations contribute to our understanding of black hole–dark matter interactions?
Answer: Computer simulations model the complex gravitational dynamics between dark matter and black holes over cosmic time. These simulations help researchers test theoretical predictions, visualize the evolution of dark matter halos, and explore how such interactions impact galactic structure and black hole growth.What theoretical models describe the interplay between dark matter and black holes?
Answer: Theoretical models often combine general relativity with particle physics to explain how dark matter particles behave in strong gravitational fields. These models predict how dark matter density can be enhanced near black holes and explore feedback mechanisms that regulate both black hole accretion and galactic evolution.How might dark matter influence the growth rate of supermassive black holes?
Answer: Dark matter can contribute to the mass inflow toward supermassive black holes by deepening the gravitational potential well. Enhanced dark matter density in galactic centers may accelerate accretion processes and influence the evolution and spin of the black hole over time.What challenges are encountered when trying to detect the direct effects of dark matter on black holes?
Answer: The primary challenges include dark matter’s weak interaction with electromagnetic radiation and the indirect nature of its gravitational effects. Distinguishing dark matter signatures from those of visible matter requires highly sensitive instruments and precise measurements of gravitational lensing and stellar dynamics.How does the presence of dark matter affect the shape and stability of galaxies?
Answer: Dark matter provides a stabilizing gravitational force that holds galaxies together, influencing their rotation curves and overall structure. Its distribution forms a halo around galaxies, which not only affects the orbits of stars but also plays a key role in the long-term evolution and stability of galactic systems.What impact do black hole–dark matter interactions have on the cosmic web?
Answer: These interactions contribute to the large-scale structure of the universe by influencing how matter clusters into filaments and voids. The gravitational interplay between dark matter and black holes affects the formation and evolution of cosmic structures, thereby shaping the overall architecture of the cosmic web.How might future research refine our understanding of the interplay between black holes and dark matter?
Answer: Future research will benefit from advancements in observational technology, such as more sensitive telescopes and gravitational wave detectors, as well as improved computer simulations. These tools will allow scientists to measure dark matter distributions more precisely and test theoretical models, ultimately deepening our understanding of these complex interactions.
Interaction Between Black Holes and Dark Matter: Thought-Provoking Questions and Answers
How might breakthroughs in dark matter detection technologies transform our understanding of black hole environments?
Answer: Breakthroughs in dark matter detection—such as ultra-sensitive detectors or novel indirect observation techniques—could provide direct evidence of dark matter particles and their distribution around black holes. This would refine our models of gravitational interactions, help calibrate computer simulations, and potentially reveal new physics governing the behavior of matter under extreme conditions.Could the interaction between black holes and dark matter offer clues toward a unified theory of quantum gravity?
Answer: Yes, studying these interactions at the intersection of astrophysics and particle physics might expose phenomena that challenge both general relativity and quantum mechanics. Insights from how dark matter behaves in the intense gravitational fields near black holes could guide the development of a theory that unifies these fundamental forces, addressing longstanding paradoxes like information loss.What might be the consequences if dark matter were found to influence the evaporation rate of black holes?
Answer: If dark matter affects black hole evaporation, it could alter the expected timescales of Hawking Radiation and mass loss. This influence might provide a new mechanism for energy exchange in black holes, affect their thermodynamic properties, and contribute to resolving the black hole information paradox, thereby reshaping our understanding of black hole lifecycle and cosmic evolution.How could advancements in computer simulations enhance our understanding of the feedback loop between dark matter and black hole growth?
Answer: Advanced simulations can model the mutual influence of dark matter and black hole accretion processes with high precision. They enable researchers to explore scenarios where dark matter density enhances black hole growth and, conversely, where energy output from black holes alters dark matter distributions. This iterative feedback loop is critical for understanding galaxy formation and evolution.What role might gravitational lensing play in directly observing dark matter distributions near black holes?
Answer: Gravitational lensing, where light from distant objects is bent by massive intervening objects, can reveal the mass distribution around black holes, including contributions from dark matter. Detailed lensing measurements can map the density and shape of dark matter halos, offering insights into how dark matter clusters and interacts with black holes in galactic cores.How could the study of black hole–dark matter interactions impact our understanding of cosmic structure formation?
Answer: By elucidating how dark matter aggregates and interacts with black holes, researchers can better understand the processes that drive the formation of galaxies and clusters. These interactions help determine the gravitational potential wells that seed cosmic structure, influencing the evolution of the cosmic web and the distribution of matter on large scales.What experimental setups or observatories might be developed to probe the interface between black holes and dark matter?
Answer: Future observatories could combine high-resolution telescopes with advanced gravitational wave detectors to capture multi-messenger data. Experimental setups might include dedicated space missions focusing on the central regions of galaxies or novel instruments designed to measure subtle gravitational lensing effects, ultimately providing detailed maps of dark matter distribution near black holes.How might variations in dark matter particle properties affect their interaction with black holes?
Answer: Different dark matter particle candidates—such as WIMPs, axions, or sterile neutrinos—would interact with gravitational fields in distinct ways. Variations in mass, cross-section, and interaction strength could lead to diverse clustering behaviors around black holes, influencing accretion rates, halo density profiles, and even the emission characteristics of nearby matter, thereby providing clues about the nature of dark matter itself.Could the study of black hole and dark matter interactions inform our search for new physics beyond the Standard Model?
Answer: Yes, anomalies in the gravitational behavior of dark matter around black holes might indicate the presence of unknown particles or forces. Such discoveries would challenge the Standard Model of particle physics and stimulate the development of new theoretical frameworks, potentially unveiling a richer tapestry of fundamental interactions that govern the universe.How might long-term observations of galactic centers refine our models of dark matter distribution in relation to black holes?
Answer: Continuous observations of galactic centers can track the dynamic evolution of dark matter halos and their interaction with central black holes. By correlating changes in stellar orbits, accretion disk activity, and gravitational lensing effects, scientists can build more accurate models of dark matter distribution, test theoretical predictions, and improve our understanding of the co-evolution of black holes and galaxies.What challenges do researchers face in disentangling the gravitational effects of dark matter from those of visible matter near black holes?
Answer: The main challenge lies in the overlapping gravitational influences of visible matter (such as stars and gas) and dark matter. High-precision measurements and sophisticated modeling are required to isolate the dark matter component. Techniques like detailed spectral analysis, high-resolution imaging, and gravitational lensing studies help differentiate these effects, yet uncertainties remain due to complex interactions in dense galactic cores.How can public outreach and interdisciplinary collaboration enhance the research on black hole–dark matter interactions?
Answer: Public outreach can increase awareness and funding for cutting-edge astrophysical research, while interdisciplinary collaboration brings together experts in astrophysics, particle physics, computational science, and engineering. These partnerships foster innovative approaches, facilitate the sharing of observational data and theoretical insights, and drive the development of new technologies that together can unlock the mysteries of how black holes and dark matter shape our universe.