Event Horizons and Singularities: The Extreme Realms of Black Holes
Among the most profound concepts in physics and astrophysics are event horizons and singularities—enigmatic features of spacetime found at the core of black holes. An event horizon is the boundary beyond which nothing, not even light, can escape the gravitational grip of a black hole. Beyond this lies a singularity, where the curvature of spacetime becomes infinite and classical physics breaks down. These ideas challenge our understanding of gravity and quantum mechanics, forming a bridge between the classical mechanics of Newton and the relativistic universe of Einstein.
Studying black holes and dark matter invites a deeper inquiry into the fundamental structure of the cosmos. Theories involving interactions between black holes and dark matter highlight how invisible forces shape galactic dynamics. In the same way, concepts like cosmology and the Big Bang Theory underscore how singularities may not only exist at the centers of black holes but also at the very origin of our universe.
The idea of a singularity raises deep theoretical problems, especially when considered alongside the universe’s earliest moments, as inferred from the cosmic microwave background and concepts like dark energy and cosmic inflation. These phenomena offer clues about the structure and evolution of the universe and the boundary conditions of spacetime itself.
Event horizons also serve as a gateway into the thermodynamics of black holes. Groundbreaking theories like Hawking radiation demonstrate that black holes are not entirely black—they emit radiation and may eventually evaporate. These findings hint at deep connections between quantum field theory and gravity, inviting novel approaches beyond the scope of traditional analytical mechanics.
Black holes form from the gravitational collapse of massive stars, making them integral to stellar physics. Their birth and remnants are closely tied to the fate of stars as explored in star life cycles and processes like nuclear fusion. The dramatic ends of stellar evolution yield compact objects such as neutron stars and white dwarfs, which are deeply connected to the conditions that produce black holes.
Exploring event horizons and singularities also enriches our understanding of the universe’s unseen components. The mysterious nature of dark matter may reveal itself more clearly through gravitational interactions near event horizons. Spectral analysis of gravitational lenses and distant stellar environments—covered in stellar spectroscopy—may eventually detect the influence of compact objects otherwise hidden from view.
From the behavior of variable stars to theoretical models involving celestial mechanics and continuum mechanics, the study of event horizons and singularities spans the full spectrum of astrophysical and physical inquiry. It exemplifies the interplay of observable phenomena with mathematical abstraction, drawing from and enriching every corner of modern science.
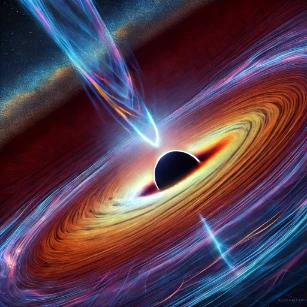
Table of Contents
Event Horizon: The Point of No Return
Definition and Nature
The event horizon is the spherical boundary surrounding a black hole. It represents the critical point beyond which nothing—not even light—can escape the immense gravitational pull of the black hole. This boundary effectively separates the black hole from the rest of the universe, cloaking the singularity and any internal activity from external observation.Key Characteristics
- No Physical Surface: The event horizon is not a physical surface but a theoretical boundary in spacetime. If an object crosses this boundary, it continues inward without experiencing any dramatic change in local conditions (assuming a sufficiently large black hole where tidal forces are weak at the horizon).
- One-Way Barrier: Information and matter can enter the event horizon but cannot escape. Once crossed, all paths forward lead inevitably toward the singularity.
- Gravitational Time Dilation: As an object approaches the event horizon, an outside observer would perceive the object slowing down due to gravitational time dilation—time slows down near intense gravitational fields. From the falling object’s perspective, however, time proceeds normally, and it crosses the event horizon without noticing any change.
- Hawking Radiation: Quantum effects near the event horizon can lead to the emission of particles, a process known as Hawking radiation. This suggests that black holes can slowly lose mass and may eventually evaporate over extremely long timescales.
Mathematical Description
The size of the event horizon depends on the mass of the black hole and is defined by the Schwarzschild radius (Rₛ) for non-rotating, uncharged black holes:Where: G is the gravitational constant M is the mass of the black hole c is the speed of light For a black hole with the mass of the Sun, the Schwarzschild radius would be about 3 kilometers (1.86 miles).
Singularity: The Heart of the Black Hole
Definition and Nature
At the core of a black hole lies the singularity, a point where matter is compressed to infinite density, and the curvature of spacetime becomes infinite. The singularity is the ultimate gravitational collapse, where the known laws of physics—both general relativity and quantum mechanics—fail to provide a coherent description.Key Characteristics
- Infinite Density and Zero Volume: All the mass of a black hole is concentrated into a point of zero volume, resulting in infinite density. This leads to a breakdown of our understanding of space and time.
- Spacetime Curvature: Near the singularity, spacetime is so severely curved that the concepts of distance and time cease to be meaningful. General relativity cannot fully describe this region due to the extreme warping.
- Breakdown of Physical Laws: Classical physics fails at the singularity. Quantum gravity—a yet-to-be-fully-developed theory—may eventually explain the behavior of matter and energy at this point.
Types of Singularities
- Point Singularity (Schwarzschild Black Hole): In a non-rotating, uncharged black hole, the singularity is a single point.
- Ring Singularity (Kerr Black Hole): In a rotating black hole, the singularity is theorized to be a ring shape due to angular momentum. Theoretically, this could allow for wormholes or even time travel, but such concepts remain speculative.
- Charged Singularity (Reissner-Nordström and Kerr-Newman Black Holes): In charged black holes, the structure of the singularity and event horizon becomes more complex, potentially including multiple horizons.
Schwarzschild Radius: The Size of the Event Horizon
Definition
The Schwarzschild radius is the critical radius at which the escape velocity equals the speed of light. Any mass compressed within this radius inevitably becomes a black hole. The Schwarzschild radius depends solely on the mass of the object:Examples of Schwarzschild Radii
- Earth: If Earth were compressed into a black hole, its Schwarzschild radius would be about 9 millimeters (about the size of a marble).
- Sun: The Sun would need to be compressed into a sphere with a radius of about 3 kilometers (1.86 miles) to become a black hole.
- Sagittarius A* (the Milky Way’s supermassive black hole): Its Schwarzschild radius is approximately 12 million kilometers (7.5 million miles).
Event Horizons in Rotating and Charged Black Holes
- Kerr Black Holes (Rotating): The event horizon is not spherical but oblate due to the black hole’s spin. A rotating black hole also has an ergosphere, where space itself is dragged around by the black hole’s rotation.
- Reissner-Nordström Black Holes (Charged): Charged black holes have two horizons: the outer event horizon and an inner Cauchy horizon. These configurations create complex causal structures, potentially allowing hypothetical wormholes.
Theoretical Implications and Paradoxes of Event Horizons and Singularities
The Information Paradox
According to quantum mechanics, information cannot be destroyed. However, anything falling into a black hole seems to be lost forever, leading to the black hole information paradox. This challenges the principles of quantum physics and has led to various theoretical proposals, including:- Holographic Principle: Suggests that all information about matter falling into a black hole is encoded on its surface (event horizon), not lost in the singularity.
- Hawking Radiation and Information Leakage: Proposes that information might gradually escape via Hawking radiation, although how this happens remains uncertain.
Cosmic Censorship Hypothesis
Proposed by Roger Penrose, this hypothesis suggests that singularities are always hidden within event horizons, preventing them from being observed. This ensures that singularities do not violate causality or the predictability of physical laws.Wormholes and Time Travel
In rotating (Kerr) and charged black holes, theoretical models suggest the possibility of wormholes—hypothetical tunnels through spacetime. However, these ideas remain speculative and face numerous physical challenges.Observational Evidence and Research in Event Horizons and Singularities
Event Horizon Telescope (EHT)
In 2019, the EHT captured the first direct image of a black hole’s event horizon in galaxy M87. The image showed the shadow of the event horizon surrounded by glowing gas, confirming theoretical predictions.Gravitational Waves
LIGO and VIRGO have detected gravitational waves from merging black holes, providing indirect evidence of event horizons and validating Einstein’s general relativity.X-ray and Radio Observations
Accretion disks and relativistic jets from material falling toward event horizons are observed in X-rays and radio waves, revealing the presence of black holes.Why Study Event Horizons and Singularities
Understanding the Boundaries of Spacetime
The event horizon marks the boundary around a black hole beyond which nothing can escape. Students learn how this concept defines the structure and behavior of black holes. It illustrates how general relativity operates under extreme conditions. Studying horizons provides insight into the nature of spacetime.
Exploring Gravitational Collapse
Singularities are points where spacetime curvature becomes infinite, indicating the limits of known physics. Students investigate how massive stars collapse to form these entities. This raises questions about quantum gravity and the fate of matter. It encourages exploration of theoretical frontiers.
Mathematical and Physical Models
Students use advanced mathematics to model horizons, geodesics, and singularity formation. These models are central to general relativity and black hole physics. Learning them strengthens problem-solving and abstract reasoning skills. It builds a solid foundation for theoretical physics.
Quantum Effects and Information Paradox
The presence of event horizons leads to questions about information loss and quantum mechanics. Students study how singularities might affect the laws of thermodynamics and causality. These issues drive major research in quantum gravity. It prepares students to tackle unresolved questions in physics.
Foundations for Future Theory Development
Event horizons and singularities point to where new theories of physics are needed. Students contribute to efforts in loop quantum gravity, string theory, and holography. This work has potential to unify general relativity and quantum mechanics. It places students at the frontier of scientific advancement.
Event Horizons and Singularities: Conclusion
The concepts of event horizons and singularities lie at the heart of our understanding of black holes, embodying some of the most extreme conditions in the universe. The event horizon marks the invisible boundary where gravity becomes inescapable, while the singularity challenges the very foundations of physics with its infinite density and curvature. Together, they push the limits of general relativity and quantum mechanics, posing profound questions about the nature of space, time, and reality itself. Ongoing research, powered by groundbreaking observations and theoretical advancements, continues to unravel these cosmic mysteries, bringing us closer to understanding the true nature of black holes and the universe.Event Horizons and Singularities: Review Questions and Answers:
What is an event horizon and why is it significant in black hole physics?
Answer: An event horizon is the boundary around a black hole beyond which nothing, not even light, can escape. It is significant because it marks the point of no return and defines the observable limits of a black hole’s influence on spacetime.What are singularities and how do they relate to black holes?
Answer: Singularities are points at the center of black holes where density becomes infinite and the laws of physics break down. They represent regions where gravitational forces crush matter into an infinitely small space, challenging our current understanding of physics.How does general relativity explain the formation of event horizons?
Answer: General relativity predicts that when a massive star collapses under its own gravity, spacetime curves so extremely that an event horizon forms. This boundary is where the escape velocity exceeds the speed of light, effectively trapping matter and radiation within.What observable phenomena indicate the presence of an event horizon?
Answer: The presence of an event horizon is inferred from the behavior of nearby matter, such as the intense radiation emitted by accretion disks, gravitational lensing effects, and the orbital motions of stars influenced by the black hole’s gravity.How do singularities challenge our current understanding of physics?
Answer: Singularities challenge our understanding by presenting conditions where density and curvature become infinite, causing conventional theories like general relativity to break down. This discrepancy highlights the need for a unified theory that reconciles gravity with quantum mechanics.What is the relationship between Hawking radiation and event horizons?
Answer: Hawking radiation is a theoretical prediction that black holes emit thermal radiation due to quantum effects near the event horizon. This phenomenon suggests that black holes can slowly lose mass and eventually evaporate, providing a bridge between quantum mechanics and gravitational theory.How do astrophysicists study regions near the event horizon despite their inaccessibility?
Answer: Astrophysicists use indirect methods such as monitoring the motion of nearby stars, analyzing emissions from accretion disks, and detecting gravitational waves from black hole mergers to study the regions near the event horizon.What role do computer simulations play in understanding singularities and event horizons?
Answer: Computer simulations model the complex gravitational interactions and spacetime dynamics near black holes. These simulations help researchers predict the behavior of matter and radiation in extreme conditions, offering insights into the physics of event horizons and singularities that cannot be observed directly.How might future observations refine our theories about event horizons and singularities?
Answer: Future observations using next-generation telescopes and gravitational wave detectors can provide higher-resolution data on black hole environments. This information could refine existing models, test predictions such as Hawking radiation, and potentially reveal new physics at the interface of general relativity and quantum mechanics.What are the theoretical implications of combining quantum mechanics with the study of event horizons?
Answer: Combining quantum mechanics with event horizon studies could lead to breakthroughs in understanding black hole thermodynamics, information loss paradoxes, and the nature of singularities. This integration is key to developing a quantum theory of gravity that unifies all fundamental forces.
Review Questions and Answers: Thought-Provoking Questions and Answers
How might breakthroughs in gravitational wave astronomy alter our understanding of event horizons?
Answer: Breakthroughs in gravitational wave astronomy could provide direct insights into the dynamics of black hole mergers and the behavior of event horizons during these cataclysmic events. By capturing the subtle ripples in spacetime, researchers can test predictions of general relativity, explore deviations that hint at quantum effects, and ultimately refine our models of black hole boundaries.What new physics might emerge from studying the interplay between quantum mechanics and event horizons?
Answer: Studying the interplay between quantum mechanics and event horizons may reveal phenomena such as quantum tunneling, fluctuations in spacetime, or even modifications to the standard model of particle physics. These insights could lead to a deeper understanding of quantum gravity and potentially uncover principles that govern the behavior of matter under extreme conditions.How could a better understanding of singularities impact the development of a unified theory of physics?
Answer: A better understanding of singularities could bridge the gap between general relativity and quantum mechanics, two pillars of modern physics that currently conflict under extreme conditions. Resolving the mysteries of singularities may pave the way for a unified theory that seamlessly integrates gravitational and quantum effects, revolutionizing our comprehension of the universe.In what ways might advanced simulations challenge or confirm our current models of event horizons?
Answer: Advanced simulations that incorporate high-resolution computational techniques and relativistic physics can model the behavior of matter near event horizons with unprecedented accuracy. These simulations can challenge current models by revealing unexpected phenomena or confirm theoretical predictions, thereby refining our understanding of black hole dynamics and the limits of classical physics.What observational strategies could be developed to detect subtle signatures of quantum effects near event horizons?
Answer: New observational strategies might include high-precision measurements of radiation spectra from accretion disks, detailed mapping of gravitational lensing effects, or the use of interferometric techniques to capture minute deviations in spacetime. Such strategies could reveal quantum fluctuations or other signatures that indicate the breakdown of classical physics near event horizons.How might the resolution of the black hole information paradox reshape our interpretation of event horizons?
Answer: Resolving the black hole information paradox could alter our interpretation of event horizons by showing how information is preserved or transformed during black hole evaporation. This breakthrough would have profound implications for the laws of thermodynamics, quantum information theory, and our overall understanding of how information interacts with gravity in extreme environments.What role could interdisciplinary research play in advancing our knowledge of singularities and event horizons?
Answer: Interdisciplinary research, combining astrophysics, quantum physics, computational science, and mathematics, can offer new perspectives and innovative methods to study singularities and event horizons. Collaborative efforts can lead to the development of novel experimental techniques, refined theoretical models, and a more comprehensive understanding of the complex physics governing black holes.How might future space missions contribute to our understanding of the regions near event horizons?
Answer: Future space missions equipped with cutting-edge telescopes and sensors could observe the high-energy emissions and gravitational effects near event horizons more precisely. Such missions may capture detailed images of the black hole shadow, measure the dynamics of accretion flows, and provide data that challenge or confirm our theoretical models of extreme spacetime.What are the potential implications of discovering deviations from classical predictions near an event horizon?
Answer: Discovering deviations from classical predictions near an event horizon could indicate the presence of new physics, such as quantum gravitational effects or unknown interactions. These findings would challenge our current theories, inspire revisions to existing models, and potentially lead to a paradigm shift in our understanding of gravity and the fundamental nature of spacetime.How could research on event horizons inform our understanding of the early universe and cosmic evolution?
Answer: Research on event horizons can inform our understanding of the early universe by shedding light on the extreme conditions that prevailed shortly after the Big Bang. Insights into black hole formation, growth, and interactions may reveal parallels with early cosmic structures, contributing to our knowledge of how matter and energy coalesced to shape the universe.What technological innovations are needed to probe the intricate physics near singularities and event horizons?
Answer: Probing the intricate physics near singularities and event horizons requires technological innovations such as ultra-sensitive detectors, next-generation space telescopes, and advanced gravitational wave observatories. These technologies would enable high-resolution observations of extreme environments, facilitate real-time data analysis, and support experimental tests of theoretical predictions in regimes where classical physics fails.How might public outreach and education initiatives inspire future generations to explore the mysteries of black holes?
Answer: Public outreach and education initiatives can demystify complex concepts like event horizons and singularities by using interactive simulations, visually engaging media, and accessible explanations. By inspiring curiosity and making advanced astrophysics relatable, these initiatives can motivate young scientists to pursue careers in research, drive public support for space exploration, and foster a deeper appreciation for the wonders of the universe.