Dark Matter: Unveiling the Hidden Universe
Dark matter represents one of the greatest mysteries in Physics and Astrophysics. Though it cannot be seen directly, its gravitational influence is evident throughout the cosmos. From the way galaxies rotate to how light bends around massive clusters, dark matter is believed to constitute approximately 85% of all matter in the universe. The study of Black Holes and Dark Matter brings together particle physics, cosmology, and gravitational theory, forming a bridge between the known and the yet-to-be-understood.
Observations of galactic rotation curves reveal discrepancies that cannot be explained by visible matter alone. This has led scientists to hypothesize the existence of dark matter, which interacts gravitationally but not electromagnetically. Its role is central in shaping the structure and evolution of the universe, alongside concepts such as dark energy and cosmic inflation, covered in topics like Multiverse and Cosmic Inflation. The foundational work of the Big Bang Theory and the Cosmic Microwave Background offer critical support for understanding how dark matter shaped early cosmological development.
While dark matter is still invisible to all known forms of electromagnetic detection, its gravitational effects are measurable. In systems involving black holes, interactions with dark matter may reveal new physics. These relationships are explored in depth in studies of black hole–dark matter interactions and in the thermodynamics of systems such as Hawking Radiation. Our comprehension of space and time is pushed further by investigating event horizons and singularities.
Dark matter’s elusive nature also has implications for Celestial Mechanics, as its mass contributes to orbital dynamics on galactic and intergalactic scales. Techniques grounded in Classical Mechanics and refined through Analytical Mechanics are employed to model these vast structures, while Continuum Mechanics contributes to simulations of matter distribution and behavior under gravitational fields.
Investigating dark matter also intersects with the study of stars. Theoretical predictions regarding stellar nucleosynthesis and the evolution of star life cycles depend on accurate models of mass and energy in galaxies. This encompasses processes such as nuclear fusion, the atmospheric analysis of stars using stellar spectroscopy, and the behavior of variable stars. Even exotic remnants like neutron stars and white dwarfs may serve as laboratories for detecting potential dark matter signals.
Ultimately, dark matter compels us to rethink the universe’s composition and origin. It continues to inspire cutting-edge experiments, ranging from particle physics to astronomical observation, as it remains one of the pillars of contemporary Physics. Its study invites collaboration across subfields, connecting stars, galaxies, and the very fabric of spacetime, providing one of the most exciting and fundamental quests in modern science.
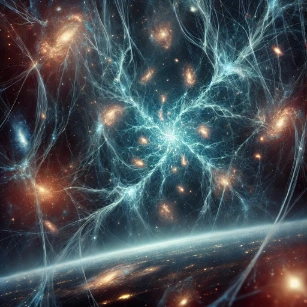
Table of Contents
1. Evidence for Dark Matter
While dark matter cannot be observed directly, its existence is inferred through multiple astrophysical observations. These indirect lines of evidence strongly suggest that a substantial amount of invisible mass permeates the cosmos.Galaxy Rotation Curves
- Observation: According to Newtonian physics, the orbital velocity of stars in a galaxy should decrease with distance from the galactic center, similar to how planets orbiting the Sun slow down with increasing distance.
- Unexpected Result: Observations by Vera Rubin and Kent Ford in the 1970s showed that stars in the outer regions of spiral galaxies rotate at unexpectedly high speeds, nearly constant with distance from the center.
- Implication: The visible matter in galaxies cannot account for these rotational speeds. A massive, unseen halo of dark matter must be providing the extra gravitational pull needed to hold galaxies together.
Gravitational Lensing
- General Relativity Prediction: Massive objects bend the fabric of spacetime, causing light from distant sources to curve around them. This effect, known as gravitational lensing, can magnify and distort background objects.
- Excessive Lensing Observed: Clusters of galaxies cause much stronger lensing than visible matter alone can explain.
- Implication: The additional mass causing the excessive lensing must be dark matter distributed throughout and around galaxy clusters.
Cosmic Microwave Background (CMB)
- CMB Fluctuations: The Cosmic Microwave Background is the remnant radiation from the Big Bang. Tiny temperature fluctuations in the CMB reflect variations in the early universe’s density.
- WMAP and Planck Observations: Measurements from the WMAP and Planck satellites reveal patterns consistent with the presence of non-luminous matter.
- Implication: The distribution and growth of these density fluctuations require dark matter to explain the formation of galaxies and cosmic structures.
Large-Scale Structure Formation
- Computer Simulations: Simulations of cosmic evolution show that visible matter alone cannot form galaxies and clusters within the universe’s current age.
- Role of Dark Matter: Dark matter provides the gravitational scaffolding necessary for baryonic matter to clump and form structures.
- Implication: The observed large-scale filamentary structure of the universe matches predictions only when dark matter is included.
The Bullet Cluster
- Observation: The Bullet Cluster is a pair of colliding galaxy clusters. Observations reveal that the hot, visible gas (detected via X-rays) is separated from most of the mass (detected via gravitational lensing).
- Implication: The separation indicates that dark matter passed through the collision unaffected, providing compelling evidence that dark matter exists independently of normal matter.
Dark Matter Candidates
Identifying the fundamental nature of dark matter is one of the greatest challenges in modern physics. Several theoretical candidates have been proposed, broadly categorized into particle-based and object-based models.Weakly Interacting Massive Particles (WIMPs)
- Description: WIMPs are hypothetical particles that interact via the weak nuclear force and gravity but not with electromagnetic radiation.
- Mass: Expected to have masses between a few GeV/c² and several TeV/c².
- Predictions: WIMPs could naturally arise from supersymmetry theories in particle physics.
- Detection Efforts: Experiments like XENON1T, LUX-ZEPLIN, and PandaX aim to detect WIMPs via direct interactions with atomic nuclei.
- Status: Despite extensive searches, WIMPs have not been detected, leading to increasingly stringent constraints on their properties.
Axions
- Description: Axions are ultra-light, hypothetical particles proposed to solve the strong CP problem in quantum chromodynamics (QCD).
- Mass: Extremely low mass (~10⁻⁶ to 10⁻³ eV/c²).
- Interaction: Weakly interacts with photons, making detection extremely challenging.
- Detection Efforts: Experiments like ADMX and CASPEr seek to detect axions through their conversion into photons in strong magnetic fields.
- Implication: Axions could make up a significant portion of dark matter.
Massive Compact Halo Objects (MACHOs)
- Description: MACHOs are large, non-luminous astrophysical objects such as brown dwarfs, black holes, or neutron stars that could populate galactic halos.
- Detection Efforts: Gravitational microlensing surveys like MACHO and EROS searched for MACHOs by observing how they bend light from background stars.
- Status: Observations suggest that MACHOs cannot account for all dark matter, although they might contribute a small fraction.
Sterile Neutrinos
- Description: Sterile neutrinos are hypothetical particles that interact only through gravity, unlike regular neutrinos, which interact via the weak nuclear force.
- Mass: Predicted to have a small but non-zero mass.
- Detection Efforts: X-ray observations look for indirect evidence of sterile neutrino decay.
- Implication: Could explain both dark matter and certain anomalies in neutrino oscillations.
Alternative Theories to Dark Matter
In the absence of direct detection, some physicists propose modifying our understanding of gravity instead of invoking dark matter.Modified Newtonian Dynamics (MOND)
- Proposal: Suggests that Newton’s laws of motion break down at extremely low accelerations, eliminating the need for dark matter.
- Challenges: While MOND explains galactic rotation curves, it fails to account for gravitational lensing and large-scale structure formation.
Emergent Gravity
- Proposal: Proposed by Erik Verlinde, suggesting gravity is not a fundamental force but an emergent phenomenon, possibly explaining dark matter-like effects.
- Status: Remains speculative and lacks empirical support.
Detection Methods and Experiments on Dark Matter
Direct Detection
- Goal: Detect dark matter particles through their rare interactions with atomic nuclei.
- Leading Experiments: XENONnT, LUX-ZEPLIN, and PandaX.
Indirect Detection
- Goal: Search for byproducts of dark matter annihilation or decay, such as gamma rays, neutrinos, or cosmic rays.
- Instruments: Fermi Gamma-ray Space Telescope, AMS-02 on the ISS.
Collider Searches
- Goal: Produce dark matter particles in high-energy collisions.
- Facilities: Large Hadron Collider (LHC) at CERN.
Dark Matter :Conclusion
Dark matter is an essential yet elusive component of the universe, shaping the formation and evolution of galaxies and large-scale cosmic structures. Despite compelling evidence for its existence through gravitational effects, its fundamental nature remains unknown. Ongoing research through direct detection, indirect observation, and collider experiments seeks to reveal this hidden mass. Solving the dark matter puzzle will not only transform astrophysics but may also unlock new realms of physics beyond the Standard Model, deepening our understanding of the universe.Why Study Dark Matter
Exploring the Invisible Universe
Dark matter is a mysterious substance that does not emit or absorb light but exerts gravitational effects. Students study how it influences galaxy rotation, gravitational lensing, and cosmic structure. Understanding dark matter reveals what makes up most of the universe’s mass. It is key to modern astrophysics and cosmology.
Techniques for Detection and Study
Students learn about indirect detection through galaxy dynamics, cosmic microwave background data, and particle detectors. This field blends observational astronomy with particle physics. It involves modeling, simulations, and data interpretation. These techniques develop technical and scientific skills.
Implications for Physics and Cosmology
Dark matter challenges existing theories and motivates extensions to the Standard Model of particle physics. Students explore how new particles or forces might explain it. This supports the development of unified physical theories. It deepens understanding of the universe’s composition and evolution.
Links to Galaxy Formation and Structure
Dark matter provides the gravitational framework for galaxy and cluster formation. Students examine how it affects the distribution of matter in the universe. This knowledge connects microscopic particles with large-scale structure. It enhances the interdisciplinary scope of physics and astronomy.
Research and Career Opportunities
Dark matter research spans astrophysics, cosmology, and high-energy physics. Students contribute to experiments in observatories, laboratories, and international collaborations. The field offers exciting career paths in academia, government, and technology sectors. It invites future discoveries and scientific impact.
Dark Matter: Review Questions and Answers:
What is dark matter and why is it significant in astrophysics?
Answer: Dark matter is a form of matter that does not emit, absorb, or reflect light, making it invisible to conventional telescopes. It is significant because its gravitational influence helps explain the motion of galaxies and the large-scale structure of the universe.How is the existence of dark matter inferred by scientists?
Answer: Scientists infer the presence of dark matter through its gravitational effects, such as the rotation curves of galaxies, gravitational lensing of background objects, and discrepancies between the observed mass and the gravitational forces acting on cosmic structures.What role does dark matter play in the formation of galaxies?
Answer: Dark matter acts as a gravitational scaffold around which galaxies form. Its presence creates potential wells that attract ordinary matter, enabling the formation and stability of galaxies and influencing their overall shape and dynamics.How do gravitational lensing studies support the dark matter hypothesis?
Answer: Gravitational lensing occurs when the gravity of a massive object, like a dark matter halo, bends light from background sources. Observations of lensing effects reveal mass distributions that cannot be explained by visible matter alone, thus supporting the existence of dark matter.What are the main theoretical candidates proposed for dark matter composition?
Answer: Theoretical candidates for dark matter include weakly interacting massive particles (WIMPs), axions, and sterile neutrinos. These candidates are hypothesized to interact primarily through gravity, making them difficult to detect using standard electromagnetic observations.How do scientists use computer simulations to study dark matter?
Answer: Computer simulations model the evolution of dark matter in the universe by simulating its gravitational interactions. These simulations help predict the formation of cosmic structures, compare theoretical models with observational data, and refine our understanding of dark matter’s role in shaping the universe.What observational techniques are used to study dark matter distribution in galaxies?
Answer: Techniques include analyzing galaxy rotation curves, measuring gravitational lensing effects, and mapping the cosmic microwave background. These methods provide indirect evidence of dark matter by revealing mass discrepancies in regions where visible matter is insufficient to explain gravitational forces.How does dark matter influence the cosmic web and large-scale structure of the universe?
Answer: Dark matter forms extensive, diffuse halos that serve as the backbone of the cosmic web. Its gravitational pull organizes matter into filaments, clusters, and voids, influencing the overall distribution of galaxies and contributing to the universe’s large-scale structure.What challenges do researchers face when trying to detect dark matter particles directly?
Answer: Direct detection of dark matter particles is challenging due to their weak interactions with ordinary matter. Experiments require extremely sensitive detectors shielded from background radiation, and despite decades of research, dark matter particles have yet to be conclusively observed in laboratory settings.What future advancements could help improve our understanding of dark matter?
Answer: Future advancements may include more sensitive detectors, improved gravitational wave observatories, and enhanced computer simulations. International collaborations and next-generation telescopes will also play a crucial role in probing dark matter’s properties and its interactions with ordinary matter.
Dark Matter: Thought-Provoking Questions and Answers
How might a breakthrough in dark matter detection transform our understanding of cosmology?
Answer: A breakthrough in dark matter detection would provide direct evidence of its particle nature, potentially validating or refuting existing theoretical models. This could revolutionize our understanding of cosmic structure formation, refine the parameters of cosmological models, and possibly unveil new physics beyond the Standard Model.What are the implications if dark matter is found to interact weakly with ordinary matter?
Answer: If dark matter is confirmed to interact weakly with ordinary matter, it would explain its elusive nature and provide insights into the fundamental forces at play. This finding could lead to the development of new detection technologies, offer clues about the early universe, and help integrate dark matter into a unified framework of particle physics.How could dark matter research influence our understanding of the fundamental laws of physics?
Answer: Research into dark matter challenges existing theories by pushing the limits of our understanding of gravity, quantum mechanics, and particle physics. Discovering its true nature may lead to revisions in the Standard Model, stimulate new theories of quantum gravity, and offer a more comprehensive picture of the forces governing the cosmos.What could be the role of dark matter in determining the fate of the universe?
Answer: Dark matter plays a crucial role in the gravitational dynamics that govern cosmic evolution. Its distribution influences the expansion rate of the universe and the formation of large-scale structures. Understanding dark matter could help predict whether the universe will continue expanding indefinitely, reach a steady state, or eventually collapse.How might new particle physics theories reconcile with dark matter observations?
Answer: New particle physics theories, such as supersymmetry or extra-dimensional models, may provide candidates for dark matter particles that align with observed gravitational effects. These theories could bridge gaps between quantum mechanics and cosmology, offering a coherent explanation for dark matter’s properties and interactions with ordinary matter.In what ways can advanced computer simulations enhance our models of dark matter distribution?
Answer: Advanced computer simulations can model the complex gravitational interactions of dark matter over cosmic timescales, predicting the formation of galaxies and cosmic filaments. These simulations allow researchers to test various dark matter candidates, compare outcomes with observational data, and refine our theoretical understanding of cosmic structure.What potential technological applications could arise from understanding dark matter properties?
Answer: Although dark matter is primarily a subject of fundamental research, breakthroughs in its understanding could lead to novel technologies in fields such as energy generation, quantum computing, or materials science. Insights into dark matter interactions might inspire innovative methods for energy storage or lead to the discovery of new particles with unique properties useful in advanced technological applications.How might dark matter affect the evolution of cosmic structures over billions of years?
Answer: Dark matter’s gravitational influence has been a constant force shaping the universe since its formation. Over billions of years, it has guided the clustering of galaxies, influenced the formation of galaxy clusters, and determined the large-scale structure of the cosmos. Its role in cosmic evolution is essential for understanding the timeline and mechanisms behind structure formation.What challenges arise in distinguishing dark matter effects from those predicted by modified gravity theories?
Answer: Distinguishing dark matter from modified gravity theories involves analyzing subtle differences in how each model explains galaxy rotation curves, gravitational lensing, and cosmic structure. The challenge lies in obtaining sufficiently precise observational data and developing robust theoretical models that can account for all observed phenomena without invoking unseen matter.How can multi-messenger astronomy contribute to our study of dark matter?
Answer: Multi-messenger astronomy, which combines data from electromagnetic signals, gravitational waves, and neutrinos, provides a more comprehensive view of cosmic events. This approach can reveal indirect effects of dark matter on various astrophysical phenomena, offering new avenues to test theories and improve our understanding of its distribution and interactions.What ethical considerations should be taken into account when prioritizing dark matter research funding?
Answer: Ethical considerations include the allocation of limited scientific resources and balancing fundamental research with practical applications that benefit society. Policymakers must consider the long-term scientific value of dark matter research, its potential to drive technological innovation, and the opportunity cost of investing in other pressing research areas.How might international collaboration accelerate breakthroughs in dark matter research?
Answer: International collaboration enables the pooling of resources, expertise, and observational data, which is crucial for tackling the complex challenges of dark matter research. Joint efforts can lead to the development of advanced detectors, coordinated global observation campaigns, and a unified theoretical framework, ultimately accelerating discoveries and fostering a deeper understanding of the universe’s most elusive components