Black Holes: An Extensive Exploration
Black holes are among the most intriguing and extreme phenomena studied in modern Physics. These cosmic objects form when massive stars collapse under the force of their own gravity, creating regions of spacetime where the gravitational pull is so strong that nothing—not even light—can escape. Studying black holes lies at the heart of Astrophysics, as they push the boundaries of our understanding of gravity, space, and time. The mathematical and physical foundations necessary to analyze such phenomena often begin with Classical Mechanics and extend through more advanced frameworks such as Analytical Mechanics and Celestial Mechanics.
At the center of every black hole lies a region of infinite density known as a singularity, surrounded by an event horizon—the boundary beyond which information cannot escape. These features continue to perplex physicists and remain key subjects in theoretical models of the universe. Groundbreaking research into Hawking Radiation and thermodynamics has added quantum mechanical perspectives to our understanding, raising profound questions about entropy, information loss, and unification theories.
The study of Stellar Physics provides the background necessary to trace how stars evolve into black holes. Processes like nuclear fusion in massive stars eventually lead to supernovae and the birth of black holes, as explained in star life cycles. Observations through stellar spectroscopy and theories of nucleosynthesis further our insight into how black holes emerge and influence their surroundings.
Many black holes are the remnants of dying stars, forming alongside other exotic objects such as neutron stars and white dwarfs. These stellar remnants play an essential role in understanding mass transfer, accretion, and the emission of high-energy radiation. The behavior of variable stars and stellar oscillations also provides valuable clues for detecting the gravitational effects of black holes in binary systems.
Black holes are central to our understanding of the larger cosmos. They are intimately tied to models of Cosmology, including the Big Bang Theory, the interpretation of the Cosmic Microwave Background, and the accelerating expansion driven by dark energy. Theoretical proposals such as cosmic inflation and the structure of the universe explore how black holes may play a formative role in the evolution of galaxies and galactic clusters.
Black holes do not exist in isolation—they are intricately connected to the dark scaffolding of the cosmos. The gravitational interplay explored in interactions between black holes and dark matter has significant implications for both astrophysical modeling and particle physics. Broadening our grasp of this relationship helps bridge the gap between the study of black holes and dark matter, offering insight into gravitational lensing, galaxy rotation curves, and large-scale structure formation.
Ultimately, the study of black holes provides a gateway into the universe’s deepest mysteries. Their behavior challenges the limits of Einstein’s relativity and beckons for unification with quantum theory. From the equations of Continuum Mechanics to the celestial choreography of orbital bodies, black holes demand a synthesis of the most fundamental ideas in science—offering students not just problems to solve, but entirely new ways to look at the universe.
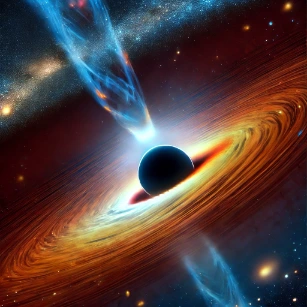
Table of Contents
Definition and Core Characteristics of Black Holes
At their core, black holes are solutions to Einstein’s General Theory of Relativity, which describes how mass and energy warp spacetime. The defining feature of a black hole is the event horizon—the boundary beyond which nothing can escape. Once matter or radiation crosses this point, it is irrevocably drawn inward toward the singularity, a region of infinite density where the known laws of physics cease to apply. Key Features of Black Holes:- Event Horizon: The invisible surface surrounding a black hole. Once crossed, escape is impossible.
- Singularity: The central point where matter is thought to collapse to infinite density.
- No Hair Theorem: Black holes are fully described by only three properties: mass, electric charge, and angular momentum (spin).
- Gravitational Time Dilation: Time slows down near the event horizon relative to an outside observer.
Types of Black Holes
Black holes are categorized based on their mass and formation mechanisms. Each type has distinct origins and characteristics that influence their role in the universe.a. Stellar-Mass Black Holes
- Mass Range: 3 to 100 times the mass of the Sun.
- Formation: Result from the gravitational collapse of massive stars (typically >20 solar masses) after exhausting their nuclear fuel and undergoing a supernova explosion.
- Characteristics:
- These are the most commonly observed black holes.
- Detected through their interaction with companion stars or surrounding matter, producing X-ray emissions.
- Example: Cygnus X-1, one of the first stellar-mass black holes discovered.
b. Supermassive Black Holes (SMBHs)
- Mass Range: Millions to billions of times the mass of the Sun.
- Location: Found at the centers of most galaxies, including the Milky Way.
- Formation Theories:
- Direct collapse of massive gas clouds in the early universe.
- Gradual growth through the accretion of matter and merging with other black holes.
- Role in Galaxies:
- Regulate star formation and galactic evolution.
- Emit powerful jets and radiation, influencing interstellar environments.
- Example: Sagittarius A*, the supermassive black hole at the Milky Way’s center.
c. Intermediate-Mass Black Holes (IMBHs)
- Mass Range: 100 to 100,000 solar masses.
- Formation (Hypothetical):
- May form from the merger of stellar-mass black holes.
- Could be remnants of Population III stars (the universe’s first stars).
- Significance:
- Potentially the “missing link” between stellar-mass and supermassive black holes.
- May reside in globular clusters or dwarf galaxies.
- Evidence: Still limited, but gravitational wave detections hint at their existence.
d. Primordial Black Holes (PBHs)
- Hypothetical: No confirmed detections yet.
- Formation: Thought to have formed in the early universe due to high-density fluctuations shortly after the Big Bang.
- Mass Range: Could range from microscopic to several solar masses.
- Cosmological Role:
- Possible contributors to dark matter.
- Could seed the growth of supermassive black holes.
Formation of Black Holes
The formation of black holes depends on complex processes driven by gravitational collapse and cosmic evolution.a. Gravitational Collapse of Massive Stars
- When a massive star (more than 20 solar masses) depletes its nuclear fuel, outward pressure from fusion reactions can no longer counteract gravity.
- The star’s core collapses under its own weight, leading to:
- Supernova Explosion: The outer layers are violently ejected.
- Core Remnant: If the core mass exceeds about 3 solar masses, it collapses into a black hole.
b. Binary Mergers and Black Hole Growth
- Stellar-mass black holes can grow by accreting matter or merging with other compact objects.
- Neutron star-black hole or black hole-black hole mergers, observed by LIGO and VIRGO, result in larger black holes and emit detectable gravitational waves.
c. Direct Collapse in Early Universe
- In the early universe, massive gas clouds could collapse directly into black holes without forming stars.
- This process may explain how supermassive black holes formed so quickly after the Big Bang.
d. Accretion and Growth
- Black holes grow by accreting nearby gas, dust, and even stars.
- Accretion disks around black holes heat up due to friction, emitting X-rays and other radiation.
Detection of Black Holes
Black holes cannot be observed directly due to their event horizon, but their presence is inferred through indirect evidence.a. X-ray Emissions
- Matter falling into a black hole forms an accretion disk that heats up and emits X-rays.
- Binary systems with black holes are identified by periodic X-ray outbursts.
b. Gravitational Lensing
- Black holes bend light from background objects, creating distorted or multiple images, a phenomenon known as gravitational lensing.
c. Gravitational Waves
- Detected by observatories like LIGO and VIRGO, gravitational waves are ripples in spacetime caused by merging black holes.
- First observed in 2015, confirming Einstein’s predictions.
d. Direct Imaging
- In 2019, the Event Horizon Telescope (EHT) captured the first image of a black hole’s shadow in M87, providing direct evidence of black holes.
Black Holes and Theoretical Physics
Black holes challenge and expand our understanding of physics.a. The Information Paradox
- According to quantum mechanics, information cannot be destroyed, but black holes appear to erase it.
- Theorized solutions include Hawking radiation and the holographic principle.
b. Hawking Radiation
- Stephen Hawking proposed that black holes emit radiation due to quantum effects near the event horizon.
- Over time, this radiation could cause black holes to evaporate.
c. General Relativity vs. Quantum Mechanics
- Black holes force physicists to reconcile Einstein’s general relativity with quantum mechanics.
- The study of singularities may lead to a unified theory of quantum gravity.
Black Holes and the Universe
Black holes play a fundamental role in shaping the cosmos.- Galactic Evolution: Supermassive black holes influence star formation and galactic dynamics.
- Cosmic Recycling: Supernovae and mergers spread heavy elements essential for planet and life formation.
- Dark Matter: Primordial black holes could account for some of the universe’s dark matter.
Black Holes Conclusion
Black holes are among the most powerful and enigmatic objects in the universe, embodying the extremes of gravity, density, and the limits of physical law. From stellar-mass black holes to supermassive giants at galactic centers, these cosmic phenomena influence galaxy formation, regulate star formation, and serve as natural laboratories for testing the most profound theories in physics. As technology and observational methods improve, the study of black holes will continue to unlock the secrets of spacetime, matter, and the fundamental workings of the universe.Why Study Black Holes
Understanding Extreme Gravity
Black holes are regions of spacetime where gravity is so intense that nothing, not even light, can escape. Students explore how black holes bend space and time, revealing the limits of general relativity. Studying them provides insights into gravitational physics and extreme environments. It enhances our understanding of cosmic laws and phenomena.
Observational Techniques and Evidence
Although invisible, black holes are studied through indirect evidence like gravitational waves and accretion disk emissions. Students learn how telescopes and detectors reveal their presence. This encourages the use of advanced technology and data analysis. It fosters innovation in astrophysical research.
Role in Galactic Evolution
Supermassive black holes are found at the centers of galaxies and influence their formation and behavior. Students study how black holes interact with stars, gas, and dust. These processes shape galaxies over billions of years. It connects black hole physics with cosmology.
Intellectual Challenge and Curiosity
Black holes challenge conventional thinking and inspire scientific curiosity. Students engage with paradoxes and open questions that push the boundaries of physics. This field nurtures critical thinking and theoretical exploration. It motivates students to ask profound questions about the universe.
Foundation for Advanced Physics Research
Studying black holes prepares students for work in general relativity, quantum gravity, and astrophysics. They develop analytical and computational skills needed for cutting-edge science. This training is relevant to research, teaching, and technological innovation. It offers a path to impactful and exciting scientific careers.
Black Holes: Review Questions and Answers:
What are black holes and how are they defined in astrophysics?
Answer: Black holes are regions in space where gravitational forces are so strong that nothing, not even light, can escape. They are defined by their event horizons—the boundaries beyond which all matter and radiation are trapped—and are key to understanding extreme gravitational phenomena.How do black holes form from stellar evolution?
Answer: Black holes typically form when massive stars exhaust their nuclear fuel and collapse under their own gravity during a supernova explosion. The core compresses to an infinitely dense point, creating a singularity surrounded by an event horizon, though alternative formation scenarios also exist.What is the event horizon and why is it significant?
Answer: The event horizon is the boundary around a black hole where the escape velocity equals the speed of light. It is significant because it marks the point of no return—once matter or radiation crosses this boundary, it can no longer influence the outside universe.How is the mass of a black hole determined?
Answer: The mass of a black hole is typically determined by observing the motion of nearby stars or gas clouds influenced by its gravitational pull. Measurements of orbital velocities and the dynamics of accretion disks allow scientists to estimate the black hole’s mass with considerable accuracy.What is Hawking radiation and what does it imply about black holes?
Answer: Hawking radiation is a theoretical prediction that black holes emit small amounts of thermal radiation due to quantum effects near the event horizon. This implies that black holes can gradually lose mass and energy over time, suggesting they are not entirely “black” but slowly evaporate.How do black holes affect the matter and energy in their vicinity?
Answer: Black holes influence nearby matter by exerting intense gravitational forces that can pull in gas and dust to form accretion disks. These disks heat up and emit high-energy radiation, while relativistic jets may also be launched, affecting the dynamics of the surrounding space.What role do accretion disks play in the study of black holes?
Answer: Accretion disks are swirling collections of matter orbiting a black hole. They are critical for studying black holes because their high-energy emissions provide indirect evidence of a black hole’s presence, and their dynamics offer insights into the physical processes occurring near the event horizon.How do black holes influence time and space around them?
Answer: The intense gravitational field of a black hole significantly warps the fabric of spacetime, causing time dilation where time appears to slow down near the event horizon relative to distant observers. This curvature affects the trajectories of nearby matter and light, providing a testing ground for theories of relativity.What observational methods are used to detect black holes?
Answer: Black holes are detected indirectly through their gravitational effects on surrounding matter. Observational methods include monitoring the motion of stars in binary systems, analyzing X-ray emissions from accretion disks, and detecting gravitational waves from black hole mergers, which all indicate the presence of these invisible objects.What challenges do scientists face when studying black holes?
Answer: Studying black holes is challenging due to their inherent invisibility and extreme environments. Difficulties include the need for indirect detection methods, the complex physics near the event horizon, reconciling quantum mechanics with gravity, and the limitations of current observational technologies.
Black Holes: Thought-Provoking Questions and Answers
How might breakthroughs in gravitational wave detection reshape our understanding of black hole mergers?
Answer: Breakthroughs in gravitational wave detection can provide unprecedented insights into the dynamics of black hole mergers by capturing the ripples in spacetime generated during these events. This data enables precise measurements of mass, spin, and energy release, offering a new way to test general relativity, probe extreme gravitational fields, and improve our understanding of cosmic evolution.What potential does the study of black holes hold for unifying quantum mechanics and general relativity?
Answer: Black holes are natural laboratories for exploring the interplay between quantum mechanics and general relativity. By studying phenomena like Hawking radiation and the behavior of matter at the event horizon, researchers hope to uncover principles that bridge the two theories, potentially leading to a unified theory of quantum gravity that resolves long-standing theoretical conflicts.How could the discovery of primordial black holes influence our understanding of the early universe?
Answer: Primordial black holes, if discovered, could provide critical clues about conditions in the early universe shortly after the Big Bang. Their existence might explain certain dark matter components, offer insights into density fluctuations in the primordial cosmos, and challenge or confirm current cosmological models, thereby enriching our understanding of the universe’s origins.What are the implications of the information paradox for black hole physics and fundamental theories?
Answer: The information paradox questions whether information that falls into a black hole is lost forever, conflicting with quantum mechanics’ requirement for information conservation. Resolving this paradox could lead to revolutionary theories that reconcile quantum mechanics with gravitational physics, potentially altering our understanding of entropy, information flow, and the very nature of reality.How do black holes affect the evolution of galaxies and their central structures?
Answer: Black holes, particularly supermassive ones at the centers of galaxies, play a crucial role in regulating star formation and galactic evolution through feedback mechanisms. Their energetic emissions and outflows can influence the interstellar medium, shape the growth of galactic bulges, and drive the co-evolution of galaxies and their central black holes over cosmic timescales.In what ways might improved simulations of black hole environments advance astrophysical theories?
Answer: Enhanced simulations using high-performance computing can model the complex physics around black holes with greater precision. These simulations help test theoretical predictions about accretion disk behavior, jet formation, and spacetime curvature, allowing researchers to refine models, predict observable phenomena, and explore scenarios that are impractical to recreate in experiments.How can the study of black hole spin and angular momentum inform our understanding of cosmic evolution?
Answer: Black hole spin and angular momentum influence the dynamics of accretion disks and jet emissions. Studying these properties provides insights into the growth history of black holes, the merger processes that affect their spin, and the transfer of angular momentum in cosmic systems, thereby shedding light on the broader mechanisms driving galaxy formation and evolution.What are the prospects for detecting black holes with next-generation telescopes and observatories?
Answer: Next-generation telescopes and observatories, equipped with advanced imaging and spectroscopy capabilities, are expected to detect finer details of black hole environments, such as the event horizon’s silhouette and dynamic interactions in accretion disks. These observations will enhance our ability to test theoretical models, study relativistic effects, and deepen our understanding of black hole physics.How might research on black holes contribute to our understanding of dark matter and dark energy?
Answer: While black holes and dark matter/dark energy are distinct phenomena, research on black holes can indirectly inform our understanding of the universe’s mass-energy composition. For instance, studying gravitational interactions in regions with high dark matter density or exploring the role of black holes in galaxy formation could provide clues about how dark matter and dark energy influence cosmic structure and expansion.What role do supermassive black holes play in the regulation of star formation within galaxies?
Answer: Supermassive black holes can regulate star formation through energetic feedback processes that heat surrounding gas and inhibit its collapse into new stars. Their outflows and radiation pressure can clear gas from galactic centers, creating a self-regulating mechanism that influences the pace of star formation and the overall evolution of the host galaxy.How can laboratory experiments and analog models contribute to testing theories about black hole physics?
Answer: Laboratory experiments and analog models, such as those using fluid dynamics or optical systems, can simulate aspects of black hole physics in controlled settings. These experiments allow researchers to observe phenomena analogous to event horizons, test predictions of Hawking radiation, and explore the interplay between quantum effects and gravity, offering valuable insights that complement astronomical observations.How might public engagement and science communication shape the future research landscape in black hole studies?
Answer: Effective public engagement and science communication can inspire the next generation of astrophysicists, secure funding for research, and promote interdisciplinary collaboration. By translating complex concepts into accessible narratives and interactive experiences, scientists can foster a broader appreciation for black hole studies, stimulate public support for space exploration, and encourage innovative approaches to unraveling the universe’s deepest mysteries.