Lorem ipsum dolor sit amet, consectetur adipiscing elit. Ut elit tellus, luctus nec ullamcorper mattis, pulvinar dapibus leo.
Black Holes and Dark Matter
Black Holes and Dark Matter represent two of the most fascinating and mysterious phenomena in the universe, both of which challenge and expand the boundaries of modern physics. These concepts are central to understanding the universe’s structure, evolution, and the fundamental laws that govern cosmic behavior. Black holes are regions where gravity is so intense that nothing, not even light, can escape their grasp, while dark matter is an invisible form of matter that exerts a gravitational pull on visible matter, influencing the formation and behavior of galaxies.
Studying these phenomena allows scientists to probe the limits of Einstein’s General Relativity, explore the intersections with quantum mechanics, and refine models of the cosmos. Black holes offer insight into extreme gravitational physics and energy dynamics, while dark matter holds the key to understanding the universe’s mass distribution and structural evolution.
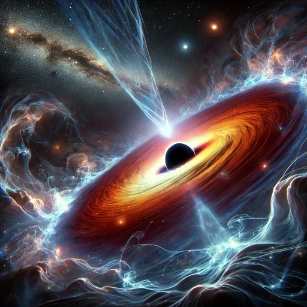
Table of Contents
Key Topics in Black Holes and Dark Matter
1. Black Holes
- Definition:
A black hole is a region of spacetime where gravity is so strong that nothing—not even light—can escape. This results from the collapse of massive stars or the merging of dense objects. - Types of Black Holes:
- Stellar-Mass Black Holes: Formed from the gravitational collapse of massive stars after supernova explosions.
- Supermassive Black Holes: Millions to billions of times the Sun’s mass, located at the centers of galaxies.
- Intermediate-Mass Black Holes: A hypothesized class between stellar and supermassive black holes.
- Primordial Black Holes: Hypothetical black holes formed in the early universe due to high-density fluctuations.
- Formation:
- Gravitational Collapse: When a star exhausts its nuclear fuel, gravity overwhelms outward pressure, causing collapse.
- Mergers: Collisions between neutron stars or black holes can produce larger black holes.
2. Event Horizons and Singularities
- Event Horizon:
The boundary around a black hole beyond which no information or matter can escape. It represents the point of no return. - Singularity:
A point at the center of a black hole where matter is infinitely dense, and space-time curvature becomes infinite. The known laws of physics break down at this point. - Schwarzschild Radius:
The critical radius at which an object becomes a black hole, dependent on its mass.
3. Hawking Radiation and Black Hole Thermodynamics
- Hawking Radiation:
Proposed by Stephen Hawking, this theoretical concept suggests that black holes emit radiation due to quantum effects near the event horizon, leading to slow evaporation over time. - Black Hole Thermodynamics:
Links gravity, quantum mechanics, and thermodynamics by assigning entropy and temperature to black holes, implying they are not entirely black but radiate energy.
4. Dark Matter
- Definition:
Dark matter is a form of matter that does not emit, absorb, or reflect light, making it invisible. However, its existence is inferred through its gravitational influence on visible matter and light in the universe. - Evidence for Dark Matter:
- Galaxy Rotation Curves: Stars in galaxies rotate faster than can be explained by visible matter alone.
- Gravitational Lensing: Light from distant galaxies bends more than expected, indicating unseen mass.
- Cosmic Microwave Background (CMB): Fluctuations in the CMB suggest the presence of non-luminous mass.
- Large-Scale Structure Formation: Simulations show that dark matter is essential for forming galaxies and clusters.
- Dark Matter Candidates:
- WIMPs (Weakly Interacting Massive Particles): Hypothetical particles that interact through gravity and weak nuclear forces.
- Axions: Ultra-light particles theorized to solve problems in quantum chromodynamics.
- MACHOs (Massive Compact Halo Objects): Dark astronomical objects like black holes, neutron stars, or brown dwarfs.
- Sterile Neutrinos: Hypothetical particles that interact only via gravity.
5. Interaction Between Black Holes and Dark Matter
- Galactic Centers:
Supermassive black holes may influence the distribution of dark matter in galactic cores. - Dark Matter Accretion:
Some theories propose that dark matter could accumulate around black holes, affecting their growth and behavior. - Primordial Black Holes as Dark Matter:
A speculative theory suggests that primordial black holes could account for a portion or all of dark matter.
Applications and Scientific Significance of Black Holes and Dark Matter
1. Probing the Limits of General Relativity and Quantum Mechanics
- Black Holes as Laboratories:
Black holes provide natural laboratories to test the limits of Einstein’s General Theory of Relativity under extreme conditions of gravity and density. - Quantum Gravity:
Understanding black hole singularities and Hawking radiation could lead to a unified theory combining quantum mechanics and general relativity.
2. Understanding Cosmic Structure Formation
- Dark Matter’s Role in Galaxy Formation:
Dark matter acts as the gravitational framework for galaxies and galaxy clusters, guiding their formation and evolution. - Gravitational Lensing:
By studying how dark matter distorts light, astronomers can map the distribution of dark matter and refine models of cosmic evolution.
3. Expanding Knowledge of the Universe’s Composition
- Dark Matter and Energy:
Combined, dark matter and dark energy make up about 95% of the universe’s total mass-energy content. Understanding dark matter is key to grasping the universe’s true composition and behavior.
4. Astrophysical Observations and Detection Techniques
- Gravitational Wave Astronomy:
Observatories like LIGO and VIRGO detect ripples in spacetime from black hole mergers, providing new ways to study these objects. - Event Horizon Telescope (EHT):
Captured the first image of a black hole’s event horizon in the galaxy M87, confirming predictions from general relativity. - Dark Matter Detectors:
Experiments like XENON1T, LUX-ZEPLIN, and AMS-02 aim to detect dark matter particles directly or indirectly.
Emerging Research and Theoretical Developments in Black Holes and Dark Matter
1. Black Hole Information Paradox
- Problem:
According to quantum mechanics, information cannot be destroyed, but black holes seem to erase information about matter they consume. - Proposed Solutions:
- Holographic Principle: Suggests that all information swallowed by a black hole is encoded on its event horizon.
- Firewalls: Hypothetical high-energy barriers at the event horizon that could resolve information loss.
2. Dark Matter Alternatives
- Modified Newtonian Dynamics (MOND):
An alternative theory suggesting that the laws of gravity behave differently at large scales, potentially eliminating the need for dark matter. - Emergent Gravity:
Proposes gravity itself might emerge from quantum information, potentially explaining dark matter-like effects.
3. Black Hole Growth and Galaxy Evolution
- Feedback Mechanisms:
Energy emitted by growing supermassive black holes can regulate star formation in galaxies. - Black Hole Mergers:
Observing gravitational waves from merging black holes reveals insights into galactic evolution.
Challenges in Studying Black Holes and Dark Matter
Direct Detection of Dark Matter:
- Despite extensive searches, dark matter particles remain undetected.
Understanding Singularities:
- Physics breaks down at black hole singularities, leaving many theoretical questions unanswered.
Observational Limitations:
- Black holes cannot be observed directly, and dark matter interacts weakly with ordinary matter, making detection challenging.
Future Directions of Black Holes and Dark Matter
Advanced Observatories:
- Next-generation telescopes like the James Webb Space Telescope (JWST) and the Euclid Space Telescope will provide deeper insights.
Gravitational Wave Astronomy:
- Facilities like LISA (Laser Interferometer Space Antenna) will detect black hole mergers and explore early-universe phenomena.
Direct Dark Matter Detection:
- Experiments such as DARWIN aim to uncover the nature of dark matter particles.
Black Hole Imaging:
- Future improvements to the Event Horizon Telescope (EHT) will capture more detailed images of black holes.
Black Holes and Dark Matter – Conclusion
Black holes and dark matter are at the forefront of modern astrophysics, pushing the boundaries of our understanding of the universe. Black holes provide critical insights into gravity, energy, and quantum physics, while dark matter shapes the very structure of the cosmos. By exploring these cosmic mysteries, scientists are not only unlocking the secrets of the universe but also paving the way for revolutionary advancements in physics and cosmology.
Black Holes and Dark Matter – Review Questions and Answers:
What are black holes and how are they defined in astrophysics?
Answer: Black holes are regions in space where gravity is so intense that nothing, not even light, can escape their pull. They are defined by their event horizons, which mark the boundary beyond which all matter and radiation are irretrievably drawn in.What is dark matter and why is it important to our understanding of the universe?
Answer: Dark matter is a form of matter that does not interact with electromagnetic radiation, making it invisible to telescopes. Its gravitational effects are essential to explain the observed motions of galaxies and the large-scale structure of the cosmos, playing a crucial role in cosmic evolution.How do black holes form in the universe?
Answer: Black holes typically form from the remnants of massive stars that have undergone a supernova explosion. When the core of such a star collapses under gravity, it can compress into a black hole, although other formation mechanisms, such as mergers and the collapse of dense gas clouds, also contribute.What observational evidence supports the existence of dark matter?
Answer: Evidence for dark matter comes from various observations, including the rotation curves of galaxies, gravitational lensing effects, and the cosmic microwave background radiation. These phenomena reveal discrepancies between the visible mass and the gravitational forces at play, implying the presence of unseen matter.How do black holes influence their surrounding environments?
Answer: Black holes affect their surroundings through their immense gravitational pull, which can draw in nearby gas and dust, forming accretion disks that emit intense radiation. They can also power high-energy jets and influence the dynamics of star clusters and galactic cores.What role does dark matter play in the formation and stability of galaxies?
Answer: Dark matter provides the necessary gravitational framework for galaxies to form and remain stable. It acts as a cosmic scaffold, attracting normal matter to form stars and galaxies while influencing the overall distribution and structure of the universe.What is the significance of an event horizon in a black hole?
Answer: The event horizon is the boundary surrounding a black hole beyond which nothing can escape its gravitational pull. It represents a point of no return, and its properties are crucial for understanding the thermodynamics, radiation, and overall behavior of black holes.What techniques are used to detect black holes if they emit no light?
Answer: Black holes are detected indirectly through their gravitational effects on nearby objects and radiation from the accretion of matter. Techniques include observing X-ray emissions from accretion disks, tracking the motion of stars orbiting invisible masses, and detecting gravitational waves from black hole mergers.How do theoretical models explain the behavior of dark matter in cosmic structures?
Answer: Theoretical models incorporate dark matter as a non-baryonic, collisionless substance that interacts primarily through gravity. These models use simulations to show how dark matter clumps together to form halos around galaxies, influencing their rotation curves and the large-scale structure of the universe.What are the current challenges in studying black holes and dark matter?
Answer: Key challenges include the inability to observe dark matter directly, understanding the complex physics near black hole event horizons, and reconciling quantum mechanics with gravitational theories. These challenges drive ongoing research and the development of new observational and theoretical tools in astrophysics.
Black Holes and Dark Matter – Thought-Provoking Questions and Answers
How might breakthroughs in detection technologies revolutionize our understanding of black holes?
Answer: Advances in detection technologies, such as improved gravitational wave detectors and high-resolution space telescopes, could reveal finer details of black hole mergers, accretion disk dynamics, and the behavior of matter near event horizons. These breakthroughs would deepen our understanding of extreme gravitational environments and potentially unveil new physics beyond current theories.What implications could a deeper understanding of dark matter have on our current cosmological models?
Answer: A more profound understanding of dark matter could refine our models of galaxy formation, cosmic evolution, and the overall structure of the universe. It might reveal whether dark matter consists of new particles or fields, potentially leading to revisions of the Standard Model of particle physics and altering our perspective on the universe’s composition.How could the study of black holes and dark matter contribute to a unified theory of physics?
Answer: Investigating black holes and dark matter pushes the boundaries of current theories, challenging our understanding of gravity, quantum mechanics, and thermodynamics. Insights gained from these studies could pave the way for a unified theory that reconciles general relativity with quantum mechanics, offering a more complete picture of the fundamental forces governing the cosmos.In what ways might improved simulations of cosmic phenomena enhance our grasp of black hole dynamics?
Answer: Enhanced simulations using high-performance computing can model the intricate interactions in black hole environments, such as accretion processes and relativistic jet formation. These simulations provide virtual laboratories to test theoretical predictions, help interpret observational data, and explore scenarios that are otherwise impossible to replicate, thereby advancing our comprehension of black hole physics.What potential discoveries could arise from exploring the interplay between dark matter and dark energy?
Answer: Exploring the interplay between dark matter and dark energy could uncover how these two elusive components interact to shape the universe’s expansion and structure. Potential discoveries might include new insights into cosmic acceleration, modifications to gravitational theory, or even the identification of new fundamental forces, all of which would revolutionize our understanding of cosmology.How might future space missions and observatories improve the detection of phenomena related to black holes and dark matter?
Answer: Future missions equipped with advanced instruments, such as next-generation X-ray observatories and gravitational wave detectors, will enhance our ability to capture high-resolution data from regions near black holes and within dark matter halos. These observatories could detect subtle signals, map dark matter distributions more accurately, and reveal dynamic processes occurring in extreme environments.What role could interdisciplinary research play in solving the mysteries of black holes and dark matter?
Answer: Interdisciplinary research, combining astrophysics, particle physics, computational science, and engineering, fosters innovative approaches to tackling complex cosmic mysteries. Collaborations across disciplines can lead to the development of new detection methods, improved theoretical models, and comprehensive data analysis techniques, thereby accelerating breakthroughs in our understanding of these phenomena.How do the concepts of information paradox and entropy challenge our current views on black hole physics?
Answer: The information paradox questions whether information that falls into a black hole is lost forever, conflicting with quantum mechanics’ principle of information conservation. This challenge forces physicists to rethink the nature of entropy, quantum information, and the fundamental laws governing black holes, potentially leading to revolutionary theories that reconcile these conflicts.What ethical and philosophical questions arise from the exploration of the universe’s most extreme phenomena?
Answer: Exploring black holes and dark matter raises questions about the limits of human knowledge, the nature of reality, and our role in the cosmos. Ethical considerations include the allocation of significant resources to fundamental research, the potential impact of discoveries on our worldview, and the responsibilities of scientists to communicate complex ideas in a manner that informs and inspires society.How might the discovery of exotic matter near black holes alter our understanding of particle physics?
Answer: Discovering exotic forms of matter near black holes—such as quark-gluon plasma or other high-energy states—could provide insights into the behavior of matter under extreme conditions. These findings may challenge or expand current particle physics models, prompting revisions to our understanding of fundamental interactions and the properties of matter at the smallest scales.What challenges do scientists face when trying to simulate the environments around black holes, and how can these be overcome?
Answer: Simulating black hole environments is challenging due to the extreme gravitational fields, complex plasma dynamics, and the need for precise numerical methods to model relativistic effects. Overcoming these challenges requires the development of sophisticated algorithms, increased computational power, and the integration of multi-physics models that can accurately capture the intricate processes occurring near event horizons.How might public engagement and education initiatives inspire the next generation of astrophysicists to explore these cosmic mysteries?
Answer: Public engagement and education initiatives can demystify complex concepts like black holes and dark matter by using interactive simulations, accessible visualizations, and storytelling techniques. By making these topics relatable and exciting, such initiatives can inspire students to pursue careers in astrophysics, drive public support for space research, and cultivate a society that values scientific inquiry and innovation.