Energy and Metabolism
Energy and metabolism are central to the functioning of all living organisms. At its core, metabolism encompasses the chemical reactions that occur within cells to maintain life—converting nutrients into usable energy and building blocks for growth and repair. These processes are governed by the laws of science, and more specifically by the principles explored in biology and physiology. Understanding metabolic processes requires familiarity with the cell biology framework in which these biochemical transformations take place.
The structure and function of cells, including the organization of cell structures and the operation of various physiological processes, are deeply tied to how energy is generated, stored, and utilized. The cell cycle and cell development are regulated by the availability of energy and the efficiency of metabolic pathways. Through cell communication and signal communication, cells coordinate their energy demands and metabolic states with their surrounding environment.
These energy transformations are also under genetic control. Insights from genetics and molecular genetics help explain how cells regulate enzyme production, balance catabolic and anabolic reactions, and respond to environmental stressors. DNA and RNA direct the synthesis of metabolic enzymes, while gene expression determines which pathways are active at any given time.
Errors in these processes—such as genetic mutations—can lead to metabolic disorders. Exploring DNA technology and applications of genetics in medicine can provide powerful tools to diagnose, understand, and treat such conditions. Research into molecular evolution and evolutionary biology also sheds light on how metabolic pathways have adapted over time across different species.
The synthesis of proteins essential for metabolism is directed by the processes described in protein synthesis, while the functional outcomes are visible in large-scale systems through mechanisms like homeostasis. The ability to maintain internal balance despite fluctuating energy intake or demand is a hallmark of life.
At a systems level, adaptation and plasticity demonstrate how metabolic networks can be reprogrammed in response to long-term changes. This is particularly evident in ecological contexts where organisms must modify their behavior and biochemistry to cope with variable conditions—a perspective enriched through the study of ecology.
Foundational topics such as molecular basis of inheritance and molecular genetics are critical for understanding how energy regulation traits are passed on, while perspectives from structure-function relationships highlight how molecular shape and dynamics affect enzymatic efficiency and transport mechanisms.
Altogether, energy and metabolism are not isolated phenomena but intricately linked with broader biological themes—from genetic encoding and protein activity to physiological regulation and evolutionary change. Mastering this topic equips students with a robust framework for tackling complex questions in biology, medicine, and environmental science.
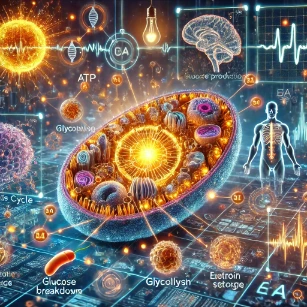
Table of Contents
Overview of Metabolism
Metabolism encompasses all chemical reactions occurring in a living organism. It is broadly divided into two main categories:
- Catabolism: The breakdown of complex molecules into simpler ones, releasing energy. Example: Cellular respiration where glucose is broken down into ATP (adenosine triphosphate).
- Anabolism: The synthesis of complex molecules from simpler ones, requiring energy input. Example: Protein synthesis from amino acids or DNA replication.
These reactions are interconnected and tightly regulated to maintain energy balance within the organism.
Sources of Energy
The primary energy sources for living organisms are:
- Carbohydrates: Broken down into glucose, the primary fuel for cellular respiration.
- Lipids (Fats): Stored as long-term energy reserves in the form of triglycerides; they yield more ATP per molecule compared to carbohydrates.
- Proteins: Used as an energy source during prolonged fasting or starvation, though their main role is structural and functional (e.g., enzymes).
Conversion of Energy: Cellular Respiration
The key process by which cells convert energy stored in food molecules into usable ATP is cellular respiration, which occurs in three main stages:
Glycolysis (in the cytoplasm)
- Glucose is broken down into two molecules of pyruvate.
- This process generates 2 ATP molecules and NADH (a high-energy electron carrier).
- Glycolysis does not require oxygen (anaerobic), allowing it to occur in all organisms.
Krebs Cycle (Citric Acid Cycle, in mitochondria)
- Pyruvate enters the mitochondria, where it is converted into acetyl-CoA.
- Acetyl-CoA is processed through the Krebs cycle, releasing CO₂ and generating NADH, FADH₂, and a small amount of ATP.
Oxidative Phosphorylation (Electron Transport Chain, in mitochondria)
- NADH and FADH₂ donate electrons to the electron transport chain in the inner mitochondrial membrane.
- Energy released by electron flow powers ATP synthesis through a process called chemiosmosis.
- Oxygen acts as the final electron acceptor, combining with hydrogen ions to form water.
- This stage produces the majority of ATP (approximately 32-34 ATP molecules).
Summary of Energy Yield: From one glucose molecule:
- Glycolysis: 2 ATP
- Krebs Cycle: 2 ATP
- Electron Transport Chain: ~32 ATP
Total Yield: ~36-38 ATP
Energy Storage and Utilization
Organisms store energy in various forms to ensure its availability when needed:
Short-term Energy Storage:
- Glucose is stored as glycogen in the liver and muscles.
- Glycogen can be quickly broken down to release glucose for immediate energy needs.
Long-term Energy Storage:
- Excess energy is stored as triglycerides (fats) in adipose tissue.
- During fasting or prolonged activity, fats are broken down into fatty acids and glycerol to produce ATP through beta-oxidation.
Energy Use During Activity:
- Immediate Energy: ATP stored in cells is used for quick, short bursts of activity (e.g., sprinting).
- Short-term Energy: Glycogen breakdown provides energy for moderate activity.
- Long-term Energy: Fat breakdown provides sustained energy during prolonged activities like endurance exercises.
Role of ATP: The Energy Currency of the Cell
ATP (adenosine triphosphate) is the primary energy carrier in cells. It consists of:
- A nitrogenous base (adenine),
- A ribose sugar,
- Three phosphate groups.
ATP stores energy in the high-energy bonds between its phosphate groups. When ATP is hydrolyzed into ADP (adenosine diphosphate) and inorganic phosphate (Pᵢ), energy is released to power cellular activities such as:
- Muscle contraction
- Active transport across cell membranes (e.g., sodium-potassium pump)
- Biosynthesis of macromolecules like proteins and nucleic acids
- Cell signaling pathways
Regulation of Metabolism
Metabolic processes are tightly regulated to balance energy production and consumption. Key mechanisms include:
- Enzymatic Regulation: Enzymes catalyze metabolic reactions and are regulated by factors such as temperature, pH, and substrate availability.
- Hormonal Control:
- Insulin promotes glucose uptake and glycogen storage after meals.
- Glucagon stimulates glycogen breakdown during fasting.
- Adrenaline triggers energy mobilization during stress or exercise.
- Feedback Inhibition: Metabolic pathways are often regulated through feedback loops where the end product inhibits earlier steps.
Energy and Metabolism in Different Organisms
- Autotrophs (e.g., plants): Convert light energy into chemical energy through photosynthesis.
- Photosynthesis generates glucose, which plants use for cellular respiration.
- Heterotrophs (e.g., animals, fungi): Obtain energy by consuming organic molecules from food.
- Anaerobic Organisms: Some organisms, like bacteria, use anaerobic respiration or fermentation when oxygen is unavailable, producing less ATP but sustaining life.
Example of Anaerobic Respiration: In humans, during intense exercise, muscles produce ATP via lactic acid fermentation when oxygen is limited.
Energy Imbalances and Their Consequences
Disruptions in energy metabolism can lead to various health problems:
- Obesity: Excessive energy intake leads to fat accumulation.
- Diabetes: Impaired glucose metabolism results from insulin dysfunction.
- Malnutrition: Insufficient energy intake hampers cellular processes and tissue repair.
- Metabolic Disorders: Conditions like mitochondrial diseases disrupt ATP production.
Importance of Energy and Metabolism
Energy and metabolism are fundamental to:
- Maintaining life processes: Energy fuels cellular activities, including growth, repair, and reproduction.
- Adapting to changes: Organisms regulate energy use during stress, exercise, or environmental fluctuations.
- Survival and evolution: Efficient metabolism ensures survival under diverse conditions, driving evolutionary success.
Why Study Energy and Metabolism
Core of Biological Function
Energy and metabolism are central to how cells perform life-sustaining processes. Students learn how organisms convert food into usable energy. These principles explain respiration, photosynthesis, and energy storage. Understanding them is essential for biology and medicine.
Biochemical Pathways and Regulation
Metabolic pathways like glycolysis and the Krebs cycle illustrate the flow of energy in cells. Students study enzymes, feedback mechanisms, and ATP production. This builds a detailed understanding of cellular efficiency. It connects chemistry to living systems.
Health and Nutritional Science
Disorders in energy metabolism can lead to obesity, diabetes, and mitochondrial diseases. Students explore how diet and hormones affect metabolism. This knowledge informs public health strategies and personal wellness. It supports careers in nutrition, healthcare, and fitness science.
Applications in Biotechnology
Metabolism is harnessed in biofuel production, fermentation, and drug development. Students learn to manipulate metabolic pathways for industrial purposes. This training supports work in biotechnology and sustainable energy. It shows how metabolism connects biology to real-world applications.
Adaptation and Metabolic Flexibility
Metabolic flexibility allows organisms to switch between fuel sources in changing conditions. Students examine how this flexibility supports survival in diverse environments. It links metabolism with adaptation and plasticity. This perspective enhances understanding of ecological and evolutionary biology.
Energy and Metabolism: Conclusion
Energy and metabolism lie at the heart of physiology, ensuring that organisms can convert food into usable energy, store it efficiently, and utilize it to power essential processes. Cellular respiration, ATP production, and metabolic regulation allow living systems to maintain homeostasis, respond to changing demands, and sustain life.Energy and Metabolism: Review Questions with Answers
Question 1: What is energy metabolism, and why is it essential for living organisms?
Answer: Energy metabolism encompasses all biochemical processes that convert nutrients into energy within living organisms. This energy is vital for maintaining cellular functions, supporting growth, enabling movement, and regulating homeostasis. Without efficient energy metabolism, cells would be unable to perform essential activities, leading to impaired function or death.
Question 2: Describe the process of glycolysis and its significance in energy production.
Answer: Glycolysis is the initial pathway of glucose catabolism, occurring in the cytoplasm of cells. It involves a series of ten enzymatic reactions that break down one molecule of glucose into two molecules of pyruvate, yielding a net gain of two ATP molecules and two NADH molecules. Glycolysis is significant because it provides quick energy without requiring oxygen and supplies intermediates for other metabolic pathways.
Question 3: How does the citric acid cycle (Krebs cycle) contribute to energy metabolism?
Answer: The citric acid cycle, also known as the Krebs cycle, takes place in the mitochondria. It processes acetyl-CoA, derived from carbohydrates, fats, and proteins, through a series of reactions that produce high-energy electron carriers: NADH and FADH₂. These carriers then donate electrons to the electron transport chain, leading to the production of ATP. The cycle also generates carbon dioxide as a waste product.
Question 4: Explain the role of the electron transport chain in ATP synthesis.
Answer: Located in the inner mitochondrial membrane, the electron transport chain (ETC) comprises a series of protein complexes that transfer electrons from NADH and FADH₂ to oxygen. This electron flow creates a proton gradient across the membrane, driving protons back through ATP synthase, an enzyme that synthesizes ATP from ADP and inorganic phosphate. This process, known as oxidative phosphorylation, produces the majority of ATP in aerobic organisms.
Question 5: What is the difference between aerobic and anaerobic metabolism?
Answer: Aerobic metabolism requires oxygen and includes processes like the citric acid cycle and oxidative phosphorylation, leading to the complete oxidation of nutrients and high ATP yield. Anaerobic metabolism occurs without oxygen, relying primarily on glycolysis, which produces less ATP and results in byproducts like lactate. While aerobic metabolism is more efficient, anaerobic pathways provide rapid energy, especially during intense or short-duration activities.
Question 6: How are fats utilized in energy metabolism?
Answer: Fats are stored as triglycerides, which are broken down into glycerol and free fatty acids. The glycerol can enter glycolysis, while fatty acids undergo beta-oxidation in the mitochondria, producing acetyl-CoA. This acetyl-CoA enters the citric acid cycle, leading to ATP production through oxidative phosphorylation. Fat metabolism provides a substantial energy source, especially during prolonged, low-intensity activities.
Question 7: What role do enzymes play in energy metabolism?
Answer: Enzymes act as biological catalysts, accelerating the chemical reactions involved in energy metabolism. They ensure that metabolic pathways proceed efficiently and are tightly regulated to meet the cell’s energy demands. For example, enzymes like hexokinase initiate glycolysis, while others like citrate synthase regulate the citric acid cycle. Enzyme activity can be modulated by factors such as substrate availability, allosteric effectors, and covalent modifications.
Question 8: How does the body regulate energy metabolism during fasting?
Answer: During fasting, the body shifts its energy metabolism to maintain blood glucose levels and supply energy to vital organs. Glycogen stores in the liver are broken down to release glucose. As fasting continues, gluconeogenesis synthesizes glucose from non-carbohydrate sources like amino acids. Additionally, increased lipolysis releases fatty acids from adipose tissue, which are converted to ketone bodies in the liver, providing an alternative energy source, particularly for the brain.
Question 9: What is the significance of the basal metabolic rate (BMR) in energy metabolism?
Answer: Basal metabolic rate (BMR) represents the amount of energy expended while at rest in a neutrally temperate environment, in the post-absorptive state. It reflects the energy required to maintain essential physiological functions such as breathing, circulation, and cellular processes. BMR accounts for the largest portion of an individual’s total daily energy expenditure and is influenced by factors like age, sex, body composition, and hormonal status.
Question 10: How do carbohydrates, fats, and proteins differ in their roles in energy metabolism?
Answer: Carbohydrates are the body’s primary and most immediate energy source, especially during high-intensity activities. They are rapidly broken down into glucose, which enters glycolysis. Fats provide a dense energy reserve, yielding more ATP per molecule through beta-oxidation and the citric acid cycle, and are predominantly utilized during prolonged, low-intensity activities. Proteins primarily serve structural and functional roles but can be catabolized into amino acids for energy production during prolonged fasting or intense exercise when other energy sources are depleted.
These questions and answers aim to deepen understanding of energy metabolism, highlighting its complexity and essential role in sustaining life.
Energy and Metabolism: Thought-Provoking Questions
Based on the topic of energy metabolism, here are 12 thought-provoking questions along with detailed and elaborate answers:
Question 1: How does the body prioritize the use of carbohydrates, fats, and proteins for energy during various intensities and durations of exercise?
Answer: The body’s energy substrate utilization depends on exercise intensity and duration:
Low-Intensity Exercise: During prolonged, low-intensity activities, the body primarily utilizes fats as the main energy source. Fatty acids are mobilized from adipose tissue and undergo beta-oxidation to produce ATP. This process is efficient for sustained energy production over extended periods.
Moderate-Intensity Exercise: As exercise intensity increases to a moderate level, there is a shift towards a greater reliance on carbohydrates. Muscle glycogen becomes a significant contributor to ATP production through glycolysis and subsequent oxidative phosphorylation. This shift allows for a more rapid supply of energy to meet the increased demands.
High-Intensity Exercise: In high-intensity, short-duration activities, the body predominantly relies on anaerobic glycolysis, rapidly breaking down muscle glycogen to lactate, yielding quick ATP but in limited amounts. This pathway does not require oxygen and provides energy swiftly, which is essential during intense efforts.
Prolonged Exercise: During extended periods of exercise, especially when glycogen stores become depleted, the body increases the utilization of fats and may also begin to catabolize proteins. Amino acids from muscle protein can be converted into intermediates that enter the citric acid cycle, contributing to energy production. However, excessive protein breakdown can lead to muscle fatigue and loss of muscle mass.
The body’s ability to adapt its energy substrate utilization based on exercise intensity and duration is crucial for optimizing performance and maintaining energy homeostasis.
Question 2: What mechanisms regulate the switch between carbohydrate and fat metabolism during rest and exercise?
Answer: The regulation of substrate utilization between carbohydrates and fats is controlled by several mechanisms:
Hormonal Regulation: Insulin and glucagon play pivotal roles. Elevated insulin levels, typically after carbohydrate ingestion, promote glucose uptake and utilization while inhibiting lipolysis. Conversely, during fasting or prolonged exercise, decreased insulin and increased glucagon levels stimulate lipolysis, enhancing fat utilization.
Allosteric Regulation: Key metabolic enzymes are regulated by the energy status of the cell. For instance, high levels of ATP inhibit phosphofructokinase, a rate-limiting enzyme in glycolysis, reducing carbohydrate metabolism. Conversely, high levels of ADP and AMP activate this enzyme, promoting glycolysis.
Substrate Availability: The availability of free fatty acids and glucose influences their utilization. High plasma free fatty acid levels favor fat oxidation, while elevated blood glucose levels promote carbohydrate utilization.
Exercise Intensity: Low-intensity exercise favors fat oxidation due to sufficient oxygen availability and slower ATP demand. As intensity increases, the demand for rapid ATP production shifts metabolism towards carbohydrates, which can be metabolized more quickly anaerobically.
These regulatory mechanisms ensure that the body efficiently switches between carbohydrate and fat metabolism to meet energy demands under varying physiological conditions.
Question 3: How does the process of gluconeogenesis maintain blood glucose levels during prolonged fasting or intense exercise?
Answer: Gluconeogenesis is the metabolic pathway that synthesizes glucose from non-carbohydrate precursors, ensuring a continuous supply of glucose during periods of fasting or intense exercise:
Precursors: The primary substrates for gluconeogenesis include lactate (produced from anaerobic glycolysis), glycerol (released from triglyceride breakdown), and glucogenic amino acids (derived from protein catabolism).
Site of Occurrence: This process predominantly occurs in the liver, with the kidneys contributing during prolonged fasting.
Regulation: Gluconeogenesis is tightly regulated by hormonal signals. Elevated glucagon and cortisol levels during fasting or stress stimulate key enzymes in the gluconeogenic pathway, while insulin inhibits them.
Physiological Importance: By producing glucose, gluconeogenesis maintains blood glucose levels within a narrow range, ensuring a constant supply of energy to glucose-dependent tissues such as the brain and red blood cells, especially when dietary intake is insufficient or during extended physical activity.
This metabolic flexibility is vital for survival during periods of limited carbohydrate availability.
Question 4: What is the role of the Cori cycle in energy metabolism, and how does it facilitate the recycling of lactate?
Answer: The Cori cycle is a metabolic pathway that connects anaerobic glycolysis in muscles with gluconeogenesis in the liver, facilitating the recycling of lactate:
Lactate Production: During intense exercise, muscles convert glucose to lactate via anaerobic glycolysis, producing ATP rapidly but also accumulating lactate, which can lead to muscle fatigue.
Lactate Transport: Lactate is transported from the muscles to the bloodstream and subsequently to the liver.
Gluconeogenesis: In the liver, lactate is converted back into glucose through gluconeogenesis.
Glucose Release: The newly synthesized glucose is released into the bloodstream, where it can be taken up by muscles and other tissues for energy production.
The Cori cycle thus plays a crucial role in maintaining energy homeostasis by recycling lactate into glucose, preventing lactate accumulation, and ensuring a continuous supply of glucose during periods of high energy demand.