Physiology
Physiology lies at the heart of understanding how living organisms function—from the molecular to the whole-organism level. It integrates knowledge from science and biology to explore dynamic processes that sustain life, including circulation, respiration, digestion, and neural signaling. As a discipline, it connects closely with cell biology and molecular genetics, translating cellular and molecular mechanisms into complex systemic behavior.
Central to physiological inquiry is the relationship between form and function, captured in topics such as structure-function relationship. Understanding how the architecture of cells and tissues supports specific roles in the body helps explain mechanisms of adaptation and efficiency. These insights are further enriched by the study of adaptation and plasticity, which examines how organisms modify their physiological responses to environmental stressors over time.
At the cellular level, physiology draws upon concepts such as cell physiology, cell structure, and cell communication. These processes underpin complex activities like signal communication, where chemical messengers and electrical impulses coordinate function across cells, tissues, and organs. These interactions are vital to achieving homeostasis, the regulation of stable internal conditions that support survival.
Physiology also encompasses energy flow within biological systems, including metabolic pathways explored in energy and metabolism. This connects with fundamental concepts in DNA and RNA processes, protein synthesis, and gene expression, which collectively dictate the production of enzymes, hormones, and structural proteins essential to bodily function.
Evolutionary insights also shape physiological studies. Topics such as evolutionary biology and molecular evolution illuminate how physiological traits have emerged and diversified over time. This includes investigating changes brought about by genetic mutation and studying broader patterns through genetics and DNA technology.
Physiological understanding is foundational to modern medicine and biotechnology. The application of discoveries from genetic research in medicine supports improved treatments, diagnostics, and health monitoring. The molecular genetics toolkit further enables researchers to dissect mechanisms of disease at the level of proteins and pathways.
Studying physiology requires integration of diverse biological processes, from the coordination of the cell cycle and cell development to the ecological interactions that shape organismal behavior, as explored in ecology. It also relies heavily on experimental tools and technologies such as those discussed in molecular techniques in research, bridging fundamental discovery with real-world application.
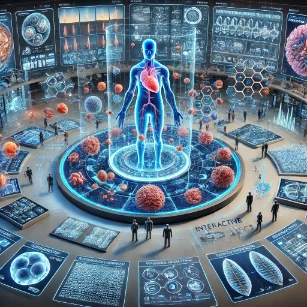
Table of Contents
Key Objectives of Physiology
Understanding Normal Biological Functions: Physiology seeks to uncover how various systems of the body function under normal conditions, forming the basis for understanding health.
Investigating Homeostasis: One of the central themes in physiology is how organisms maintain stable internal environments despite fluctuations in external conditions, ensuring survival and optimal function.
Exploring Mechanisms of Adaptation: Physiology examines how organisms adapt to changing environments, such as how the body responds to exercise, altitude, or temperature changes.
Advancing Medical Science: Physiology provides the foundation for understanding diseases, enabling the development of diagnostic tools, treatments, and preventive strategies.
Integrating Biological Levels: Physiology connects processes at the molecular and cellular levels to the functioning of organs, systems, and the entire organism.
Levels of Study in Physiology
Cellular Physiology: Focuses on the functions of cells, the basic units of life, including processes such as energy production, signal transduction, and membrane transport.
Organ Physiology: Examines the specific functions of organs, such as the heart, lungs, and kidneys, and how they contribute to overall health and function.
Systemic Physiology: Studies how organ systems, such as the circulatory, respiratory, and nervous systems, work together to maintain homeostasis and enable complex behaviors.
Comparative Physiology: Investigates the similarities and differences in physiological processes across different species, providing insights into evolution and adaptation.
Pathophysiology: Explores how normal physiological processes are altered in diseases, helping to bridge basic science and clinical medicine.
Environmental Physiology: Examines how organisms interact with and adapt to their external environments, such as extreme temperatures or oxygen-deprived conditions.
Major Themes in Physiology
Homeostasis: A cornerstone of physiology, homeostasis refers to the body’s ability to maintain a stable internal environment through feedback mechanisms. Examples include the regulation of blood pressure, body temperature, and pH balance.
Energy and Metabolism: Physiology studies how organisms convert energy from food into usable forms, store it, and use it to power cellular and systemic functions.
Signal Transmission and Communication: This includes how cells communicate through electrical signals (as in the nervous system) and chemical signals (hormones and neurotransmitters).
Structure-Function Relationship: Physiology emphasizes the connection between biological structures and their functions, such as how the shape of red blood cells optimizes oxygen transport.
Adaptation and Plasticity: Physiological processes are not static; they adapt over time. For example, the cardiovascular system adjusts during exercise, and the body acclimates to high altitudes.
Applications of Physiology
Medicine and Healthcare:
- Physiology forms the scientific basis for understanding and treating diseases, including hypertension, diabetes, and neurodegenerative disorders.
- It is central to medical diagnostics, such as interpreting electrocardiograms (ECGs) or pulmonary function tests.
Exercise and Sports Science:
- Physiology explains how physical activity affects the body and guides the development of training programs and strategies to enhance athletic performance.
Pharmacology:
- Physiology underpins the development and testing of drugs by elucidating how they interact with biological systems.
Environmental and Space Biology:
- Understanding physiological adaptation helps improve human performance and safety in extreme environments, such as deep-sea diving or space exploration.
Agriculture and Veterinary Science:
- Physiology aids in improving animal health and productivity in agriculture and provides insights into the care of domesticated and wild animals.
Historical Development of Physiology
The history of physiology dates back to ancient civilizations, where early physicians and philosophers speculated about the nature of life. Key milestones include:
Ancient Foundations:
- Hippocrates (c. 460–370 BCE) emphasized the importance of balance in bodily fluids, forming the basis of humoral theory.
- Galen (129–216 CE) conducted early experiments on animal physiology and laid the groundwork for understanding organ function.
The Scientific Revolution:
- William Harvey (1578–1657) discovered the circulation of blood, marking the beginning of modern physiology.
- The invention of the microscope in the 17th century allowed scientists like Antonie van Leeuwenhoek to observe cells and microorganisms.
19th Century Advances:
- Claude Bernard (1813–1878) introduced the concept of the internal environment (milieu intérieur) and the idea of homeostasis.
- The development of experimental methods and instruments revolutionized the study of physiological processes.
20th and 21st Century Innovations:
- Advances in molecular biology and genetics have deepened our understanding of physiology at the cellular and molecular levels.
- Modern imaging techniques, such as MRI and PET scans, have enabled non-invasive studies of organ function in real-time.
The Future of Physiology
Physiology continues to be a dynamic and evolving field, driven by technological advancements and interdisciplinary approaches. Areas of growth include:
Systems Biology: Integrating computational models with experimental data to understand complex biological systems as a whole.
Personalized Medicine: Using physiological insights to tailor treatments based on an individual’s genetic, environmental, and lifestyle factors.
Synthetic Biology: Designing and engineering biological systems with novel functions, guided by principles of physiology.
Aging and Longevity: Investigating the physiological processes of aging to develop interventions that promote healthy aging and extend life span.
Global Health Challenges: Addressing issues such as metabolic disorders, pandemics, and environmental health risks through physiological research.
Why Study Physiology
Understanding Body Functions
Physiology examines how organs, tissues, and systems work together to sustain life. Students learn how the body regulates temperature, pH, fluids, and metabolism. This knowledge is fundamental for biology, medicine, and health sciences. It provides a systems-level view of human and animal biology.
Foundations of Health and Disease
Disruptions in physiological functions lead to illness and disease. Students studying physiology understand the mechanisms behind conditions like hypertension, diabetes, and asthma. This insight supports diagnosis, treatment, and prevention strategies. It is essential for careers in healthcare and biomedical research.
Link to Biochemistry and Anatomy
Physiology connects chemical processes with structural anatomy to explain body functions. Students explore how enzymes, hormones, and nutrients interact with organs. This integrated approach enhances their understanding of biological systems. It bridges molecular biology with real-world functions.
Experimental and Diagnostic Techniques
Studying physiology involves learning to measure vital signs, analyze blood samples, and interpret test results. Students gain hands-on experience in labs using modern diagnostic tools. These skills are valuable in clinical, academic, and research environments. Practical training enhances both learning and career readiness.
Applications in Sports, Fitness, and Nutrition
Physiology is key to optimizing athletic performance, recovery, and nutritional planning. Students understand how training and diet affect muscle function, oxygen delivery, and energy balance. This field offers careers in sports science, wellness, and rehabilitation. It also promotes personal health and fitness awareness.
Physiology:Summary
In summary, physiology is a cornerstone of life sciences and medicine, offering deep insights into the intricate mechanisms that sustain life. Its emphasis on understanding normal function and adaptation makes it essential for addressing human health challenges, advancing medical science, and exploring the frontiers of biology. As we continue to unravel the mysteries of life, physiology will remain at the forefront, illuminating the dynamic processes that enable organisms to thrive in an ever-changing world.Physiology Review Questions and Answers:
Question 1 (Homeostasis)
What is homeostasis, and why is it crucial for the proper functioning of living organisms?
Answer:
Homeostasis Defined: Homeostasis is the process by which living organisms maintain a stable internal environment despite external changes. It involves the regulation of various physiological parameters such as temperature, pH, glucose levels, and electrolyte balance to ensure optimal functioning of cells and organs.
Importance of Homeostasis:
- Optimal Cellular Function:
- Enzyme Activity: Enzymes, which catalyze biochemical reactions, function best within specific temperature and pH ranges. Homeostasis ensures these conditions are maintained, facilitating efficient metabolism.
- Survival and Adaptation:
- Environmental Changes: Organisms are constantly exposed to fluctuating external conditions. Homeostasis allows them to adapt and survive by regulating internal conditions.
- Coordination of Body Systems:
- Integrated Processes: Homeostasis requires the coordination of multiple body systems (e.g., nervous and endocrine systems) to respond to internal and external stimuli effectively.
- Prevention of Disease:
- Balanced Physiology: Disruptions in homeostasis can lead to diseases. For example, impaired glucose regulation can result in diabetes, highlighting the necessity of maintaining balance.
- Energy Efficiency:
- Resource Allocation: Maintaining homeostasis ensures that energy is used efficiently, preventing wasteful processes and promoting overall health.
Mechanisms of Homeostasis:
- Feedback Systems:
- Negative Feedback: Most homeostatic mechanisms rely on negative feedback, where a change in a parameter triggers a response that counteracts the initial change (e.g., body temperature regulation).
- Positive Feedback: Less common, positive feedback amplifies changes (e.g., blood clotting).
- Sensors, Integrators, and Effectors:
- Sensors: Detect changes in the environment (e.g., thermoreceptors sensing temperature).
- Integrators: Process information and determine the appropriate response (e.g., the hypothalamus).
- Effectors: Execute responses to restore balance (e.g., sweat glands to cool the body).
Examples of Homeostatic Regulation:
Temperature Regulation:
- Scenario: When body temperature rises, sweat glands are activated to produce sweat, cooling the body through evaporation.
- Negative Feedback Loop: Temperature sensors in the skin detect the rise, the brain (integrator) signals sweat production (effector), reducing body temperature.
Blood Glucose Regulation:
- Scenario: After eating, blood glucose levels increase.
- Hormonal Control: The pancreas secretes insulin, facilitating glucose uptake by cells, thereby lowering blood glucose levels.
- Negative Feedback Loop: Elevated glucose is sensed, insulin is released to reduce it, maintaining balance.
Conclusion:
Homeostasis is essential for the survival and efficient functioning of living organisms. By maintaining internal stability through intricate regulatory mechanisms, organisms can adapt to external changes, ensure optimal cellular function, and prevent disease. Understanding homeostasis provides foundational insights into physiology and the interconnectedness of body systems.
Question 2 (Nervous System)
Describe the structure and function of the human nervous system, highlighting the differences between the central and peripheral nervous systems.
Answer:
Human Nervous System Overview: The human nervous system is a complex network responsible for coordinating voluntary and involuntary actions, transmitting signals between different parts of the body, and facilitating cognition, emotion, and memory. It is broadly divided into two main components: the Central Nervous System (CNS) and the Peripheral Nervous System (PNS).
Central Nervous System (CNS):
Structure:
- Brain: The control center of the body, responsible for processing sensory information, initiating motor commands, and enabling cognitive functions like thinking, memory, and emotion.
- Spinal Cord: A long, thin structure extending from the brain, serving as a conduit for signals between the brain and the rest of the body. It also coordinates reflexes independently of the brain.
Function:
- Information Processing: Integrates sensory data received from the body and the environment, making decisions and generating appropriate responses.
- Motor Control: Sends commands to muscles and glands to execute movements and physiological processes.
- Higher Functions: Facilitates complex behaviors, problem-solving, language, and consciousness.
Peripheral Nervous System (PNS):
Structure:
- Nerves: Bundles of axons extending from the CNS to various parts of the body, including muscles, organs, and skin.
- Ganglia: Clusters of neuronal cell bodies located outside the CNS, acting as relay points for nerve signals.
Subdivisions:
- Somatic Nervous System: Controls voluntary movements and transmits sensory information from the skin and muscles to the CNS.
- Autonomic Nervous System (ANS): Regulates involuntary functions such as heart rate, digestion, and respiratory rate. It is further divided into:
- Sympathetic Nervous System: Prepares the body for ‘fight or flight’ responses during stress or emergencies.
- Parasympathetic Nervous System: Promotes ‘rest and digest’ activities, conserving energy and maintaining homeostasis.
Differences Between CNS and PNS:
Aspect | Central Nervous System (CNS) | Peripheral Nervous System (PNS) |
---|---|---|
Components | Brain and spinal cord | Nerves and ganglia outside the CNS |
Function | Information processing and integration | Signal transmission to and from the CNS |
Protection | Encased in the skull and vertebral column; protected by cerebrospinal fluid and meninges | Less protected; surrounded by connective tissue |
Regeneration Capacity | Limited ability to regenerate | Some capacity for regeneration, especially in peripheral nerves |
Control | Higher-level control (cognition, emotions) | Both voluntary (somatic) and involuntary (autonomic) control |
Examples of CNS and PNS Functions:
CNS Function Example:
- Processing Visual Information: The brain’s occipital lobe receives and interprets signals from the eyes, enabling vision and recognition of objects.
PNS Function Example:
- Reflex Action: The spinal cord processes a reflex action, such as withdrawing a hand from a hot surface, without involving the brain for immediate response.
Clinical Relevance:
CNS Disorders:
- Examples: Stroke, multiple sclerosis, Parkinson’s disease.
- Impact: Affect information processing, motor control, and cognitive functions.
PNS Disorders:
- Examples: Peripheral neuropathy, Guillain-Barré syndrome.
- Impact: Affect signal transmission to and from the limbs and organs, leading to muscle weakness, pain, or sensory deficits.
Conclusion: The human nervous system, comprising the CNS and PNS, orchestrates the body’s responses to internal and external stimuli, ensuring coordinated and adaptive functioning. Understanding the distinct structures and roles of the CNS and PNS is fundamental to comprehending how the body maintains homeostasis, performs complex tasks, and responds to challenges. This knowledge is also crucial for diagnosing and treating neurological disorders that disrupt these intricate systems.
Question 3 (Synaptic Transmission)
Explain the process of synaptic transmission in neurons, including the roles of neurotransmitters and synaptic vesicles.
Answer:
Synaptic Transmission Overview: Synaptic transmission is the process by which neurons communicate with each other or with effector cells (such as muscle or gland cells). This communication occurs at specialized junctions called synapses, where the axon terminal of a presynaptic neuron interacts with the dendrite or cell body of a postsynaptic neuron.
Key Components:
- Presynaptic Neuron:
- Axon Terminal: The end portion of the neuron that releases neurotransmitters.
- Synaptic Vesicles: Small, membrane-bound sacs within the axon terminal that store neurotransmitters.
- Postsynaptic Neuron:
- Receptors: Protein structures on the dendrites or cell body that bind neurotransmitters, initiating a response.
- Synaptic Cleft:
- Gap: The small space between the presynaptic and postsynaptic neurons through which neurotransmitters diffuse.
Steps of Synaptic Transmission:
- Action Potential Arrival:
- An electrical impulse (action potential) travels down the axon of the presynaptic neuron and reaches the axon terminal.
- Calcium Influx:
- Voltage-Gated Calcium Channels: The depolarization caused by the action potential opens these channels, allowing Ca²⁺ ions to enter the presynaptic terminal.
- Neurotransmitter Release:
- Vesicle Fusion: The influx of Ca²⁺ ions triggers synaptic vesicles to move toward and fuse with the presynaptic membrane.
- Exocytosis: Neurotransmitters are released into the synaptic cleft through this fusion process.
- Neurotransmitter Binding:
- Receptor Interaction: Released neurotransmitters diffuse across the synaptic cleft and bind to specific receptors on the postsynaptic neuron.
- Postsynaptic Response:
- Ion Channel Activation: Binding of neurotransmitters can open or close ion channels in the postsynaptic membrane, leading to changes in the postsynaptic cell’s membrane potential.
- Excitatory Postsynaptic Potential (EPSP): Depolarizes the postsynaptic membrane, increasing the likelihood of an action potential.
- Inhibitory Postsynaptic Potential (IPSP): Hyperpolarizes the postsynaptic membrane, decreasing the likelihood of an action potential.
- Neurotransmitter Clearance:
- Reuptake: Transporters on the presynaptic neuron reabsorb neurotransmitters from the synaptic cleft for reuse.
- Enzymatic Degradation: Enzymes break down neurotransmitters in the synaptic cleft.
- Diffusion: Neurotransmitters diffuse away from the synaptic cleft, terminating their action.
Roles of Neurotransmitters and Synaptic Vesicles:
Neurotransmitters:
- Chemical Messengers: Serve as the medium for transmitting signals between neurons.
- Types: Include excitatory neurotransmitters (e.g., glutamate), inhibitory neurotransmitters (e.g., GABA), and modulatory neurotransmitters (e.g., dopamine, serotonin).
- Function: Determine the nature of the postsynaptic response based on receptor type and neurotransmitter properties.
Synaptic Vesicles:
- Storage: Contain and protect neurotransmitters within the presynaptic neuron.
- Release Mechanism: Undergo a cycle of docking, priming, fusion, and recycling to ensure efficient neurotransmitter release.
- Regulation: Their release is tightly controlled by Ca²⁺ levels and SNARE proteins that mediate vesicle fusion with the membrane.
Clinical Relevance:
Neurological Disorders:
- Alzheimer’s Disease: Involves deficits in neurotransmitter systems, particularly acetylcholine.
- Parkinson’s Disease: Characterized by reduced dopamine levels.
- Depression: Linked to imbalances in serotonin and norepinephrine.
Pharmacological Interventions:
- Antidepressants: Often target serotonin reuptake mechanisms to increase serotonin availability in the synaptic cleft.
- Anesthetics: Can interfere with synaptic transmission to inhibit neuronal activity.
Conclusion: Synaptic transmission is a fundamental process that underlies neuronal communication and the functioning of the nervous system. By meticulously orchestrating the release, binding, and clearance of neurotransmitters, neurons can transmit signals rapidly and precisely, enabling everything from reflexes to complex cognitive functions. Disruptions in synaptic transmission can lead to various neurological disorders, highlighting the critical importance of understanding this process in both health and disease.
Question 4 (Cardiovascular Sstem)
Discuss the structure and function of the human cardiovascular system, emphasizing the roles of the heart, blood vessels, and blood.
Answer:
Human Cardiovascular System Overview: The cardiovascular system, also known as the circulatory system, is responsible for transporting blood, nutrients, oxygen, carbon dioxide, hormones, and waste products throughout the body. It consists of the heart, blood vessels, and blood, each playing a critical role in maintaining homeostasis and supporting bodily functions.
Components of the Cardiovascular System:
Heart:
Structure:
- Chambers: The heart has four chambers—two atria (upper chambers) and two ventricles (lower chambers).
- Valves: Four main valves (tricuspid, pulmonary, mitral, and aortic) regulate blood flow and prevent backflow.
- Layers: Composed of three layers—endocardium (inner), myocardium (middle, muscular layer), and pericardium (outer protective sac).
Function:
- Pumping Blood: The heart acts as a pump, circulating blood through two main circuits: systemic circulation (to the body) and pulmonary circulation (to the lungs).
- Oxygenation: Facilitates the exchange of oxygen and carbon dioxide by pumping deoxygenated blood to the lungs and oxygenated blood to the body.
- Maintaining Blood Pressure: Generates the force necessary to propel blood through the circulatory system, ensuring adequate tissue perfusion.
Blood Vessels:
Types and Structure:
- Arteries:
- Function: Carry oxygenated blood away from the heart (except for pulmonary arteries, which carry deoxygenated blood to the lungs).
- Structure: Thick, elastic walls composed of three layers (tunica intima, tunica media, tunica externa) to withstand high pressure.
- Veins:
- Function: Return deoxygenated blood to the heart (except for pulmonary veins, which carry oxygenated blood from the lungs).
- Structure: Thinner walls with larger lumens compared to arteries; contain valves to prevent backflow.
- Capillaries:
- Function: Facilitate the exchange of gases, nutrients, and waste products between blood and tissues.
- Structure: Microscopic, single-cell-thick walls that allow for efficient diffusion.
Function of Blood Vessels:
- Transport: Serve as conduits for blood flow, ensuring the distribution of essential substances and removal of metabolic wastes.
- Regulation: Blood vessels can constrict or dilate to regulate blood pressure and direct blood flow to different body regions based on demand.
- Protection: Arteries and veins are reinforced to handle the mechanical stresses of blood circulation, while capillaries provide a delicate interface for exchange processes.
- Arteries:
Blood:
Components:
- Plasma:
- Composition: Approximately 55% of blood volume, consisting of water, electrolytes, proteins (albumin, globulins, fibrinogen), hormones, and waste products.
- Function: Acts as a medium for transporting nutrients, hormones, and waste; maintains blood pressure and pH balance.
- Red Blood Cells (Erythrocytes):
- Structure: Biconcave, disc-shaped cells lacking nuclei, containing hemoglobin.
- Function: Transport oxygen from the lungs to tissues and facilitate carbon dioxide removal from tissues to the lungs.
- White Blood Cells (Leukocytes):
- Types: Includes neutrophils, lymphocytes, monocytes, eosinophils, and basophils.
- Function: Part of the immune system, defending against infections, foreign invaders, and abnormal cells.
- Platelets (Thrombocytes):
- Function: Essential for blood clotting and wound repair, preventing excessive bleeding.
- Plasma:
Circulatory Pathways:
- Systemic Circulation:
- Route: Oxygenated blood is pumped from the left ventricle through the aorta to the body’s tissues and returns deoxygenated blood to the right atrium via the superior and inferior vena cavae.
- Pulmonary Circulation:
- Route: Deoxygenated blood is pumped from the right ventricle through the pulmonary arteries to the lungs for oxygenation and returns oxygenated blood to the left atrium via the pulmonary veins.
Regulation of the Cardiovascular System:
- Heart Rate and Force:
- Autonomic Nervous System: Sympathetic stimulation increases heart rate and contractility, while parasympathetic stimulation decreases heart rate.
- Blood Vessel Tone:
- Vasoconstriction and Vasodilation: Regulated by neural signals and hormones (e.g., adrenaline causes vasoconstriction in some vessels and vasodilation in others), affecting blood pressure and flow distribution.
- Blood Volume and Composition:
- Kidneys and Hormones: Regulate blood volume through fluid balance mechanisms involving hormones like aldosterone and antidiuretic hormone (ADH).
Clinical Relevance:
- Cardiovascular Diseases:
- Examples: Hypertension, atherosclerosis, heart failure, arrhythmias.
- Impact: Affect the efficiency and functionality of the cardiovascular system, leading to serious health complications.
- Blood Disorders:
- Examples: Anemia (reduced red blood cells), leukemia (abnormal white blood cells), thrombocytopenia (low platelet count).
- Impact: Impair oxygen transport, immune function, and blood clotting processes.
Conclusion: The human cardiovascular system is integral to sustaining life by ensuring the continuous transport of essential substances and the removal of wastes. The coordinated function of the heart, blood vessels, and blood facilitates efficient circulation, maintaining homeostasis and supporting the body’s diverse physiological needs. Understanding the structure and function of each component provides foundational knowledge for diagnosing and treating cardiovascular and blood-related disorders, emphasizing the system’s critical role in overall health.
Question 5 (Oxygen Transport Process)
Describe the process of oxygen transport in the blood, including the role of hemoglobin and the factors that influence oxygen binding and release.
Answer:
Oxygen Transport Overview: Oxygen transport in the blood is essential for cellular respiration, where cells utilize oxygen to produce energy in the form of ATP. This process relies on the efficient binding, transport, and release of oxygen molecules, primarily facilitated by hemoglobin within red blood cells.
Role of Hemoglobin:
- Structure of Hemoglobin:
- Composition: Hemoglobin is a tetrameric protein composed of four subunits, each containing a heme group bound to an iron ion (Fe²⁺).
- Heme Groups: Each heme group can bind one oxygen molecule (O₂), allowing each hemoglobin molecule to carry up to four oxygen molecules.
- Function of Hemoglobin:
- Oxygen Binding: In the lungs, where oxygen concentration is high, hemoglobin binds oxygen molecules, forming oxyhemoglobin.
- Oxygen Release: In tissues, where oxygen concentration is lower, hemoglobin releases oxygen molecules for cellular use.
- Carbon Dioxide Transport: Hemoglobin also plays a role in transporting carbon dioxide (CO₂) from tissues to the lungs for exhalation, though this involves different binding sites and mechanisms.
Mechanism of Oxygen Binding and Release:
Oxygen Binding:
- High Oxygen Concentration: In the pulmonary capillaries, the high partial pressure of oxygen (pO₂) favors the binding of O₂ to hemoglobin.
- Conformational Change: Oxygen binding induces a conformational change in hemoglobin from the T (tense) state to the R (relaxed) state, increasing its affinity for additional oxygen molecules.
Oxygen Release:
- Low Oxygen Concentration: In the systemic capillaries, the lower pO₂ facilitates the release of O₂ from hemoglobin to the surrounding tissues.
- Conformational Reversion: The loss of oxygen causes hemoglobin to revert to the T state, decreasing its affinity for oxygen and promoting further release.
Factors Influencing Oxygen Binding and Release:
Partial Pressure of Oxygen (pO₂):
- Direct Influence: Higher pO₂ enhances oxygen binding, while lower pO₂ promotes release.
pH Level (Bohr Effect):
- Acidosis (Lower pH): Promotes oxygen release by decreasing hemoglobin’s affinity for oxygen.
- Alkalosis (Higher pH): Enhances oxygen binding by increasing hemoglobin’s affinity for oxygen.
Carbon Dioxide Levels (Bohr Effect):
- Increased CO₂: Leads to lower pH and promotes oxygen release.
- Decreased CO₂: Results in higher pH and enhances oxygen binding.
Temperature:
- Higher Temperature: Facilitates oxygen release by decreasing hemoglobin’s affinity.
- Lower Temperature: Promotes oxygen binding by increasing hemoglobin’s affinity.
2,3-Bisphosphoglycerate (2,3-BPG):
- Function: Binds to hemoglobin, stabilizing the T state and promoting oxygen release.
- Adaptation: Elevated levels of 2,3-BPG (e.g., at high altitudes) enhance oxygen unloading to tissues.
Altitude:
- Low Altitude: Higher oxygen availability increases hemoglobin’s oxygen loading.
- High Altitude: Reduced oxygen availability necessitates mechanisms to enhance oxygen release to compensate.
Clinical Relevance:
Anemia:
- Condition: Reduced hemoglobin levels decrease the blood’s oxygen-carrying capacity.
- Impact: Can lead to fatigue, weakness, and impaired organ function due to insufficient oxygen delivery.
Carbon Monoxide Poisoning:
- Mechanism: Carbon monoxide (CO) binds to hemoglobin with higher affinity than oxygen, displacing oxygen and reducing oxygen transport.
- Impact: Causes tissue hypoxia, leading to symptoms like headache, dizziness, and in severe cases, death.
Respiratory Disorders:
- Impact: Conditions like chronic obstructive pulmonary disease (COPD) can impair oxygen uptake in the lungs, affecting overall oxygen transport and delivery.
Conclusion: Oxygen transport in the blood is a finely tuned process, intricately regulated by hemoglobin and influenced by various physiological factors. Understanding the dynamics of oxygen binding and release, along with the factors that modulate hemoglobin’s affinity for oxygen, is essential for comprehending how the body meets its metabolic demands under varying conditions. This knowledge is also critical for diagnosing and managing disorders related to impaired oxygen transport, ensuring effective interventions to maintain cellular and overall health.
Question 6 (Autonomic Nervous System)
Explain the mechanisms of action for the sympathetic and parasympathetic divisions of the autonomic nervous system, and describe how they maintain homeostasis in the body.
Answer:
Autonomic Nervous System (ANS) Overview: The autonomic nervous system is a subdivision of the peripheral nervous system responsible for regulating involuntary physiological functions, including heart rate, digestion, respiratory rate, pupillary response, and more. It operates largely below the level of conscious control and is divided into two main branches: the sympathetic and parasympathetic nervous systems.
Sympathetic Nervous System (SNS):
Mechanism of Action:
- Neurotransmitters: Primarily uses norepinephrine (noradrenaline) as its main neurotransmitter at target organs.
- Receptors: Acts on adrenergic receptors (α and β subtypes) located on effector organs.
- Ganglia: Consists of a network of sympathetic ganglia near the spinal cord, with preganglionic neurons releasing acetylcholine to stimulate postganglionic neurons.
Physiological Effects:
- Fight or Flight Response: Prepares the body for emergency situations by increasing alertness and energy expenditure.
- Cardiovascular System: Increases heart rate and contractility, dilates bronchioles, and constricts blood vessels in non-essential areas.
- Respiratory System: Dilates airways to enhance oxygen intake.
- Metabolic Effects: Stimulates glycogenolysis and lipolysis to provide energy.
- Digestive System: Inhibits digestive activities, redirecting energy to more critical functions.
- Pupillary Response: Causes pupil dilation (mydriasis) to improve vision.
Role in Homeostasis:
- Adaptation to Stress: Balances the body’s immediate needs during stress or physical exertion, ensuring survival.
- Energy Mobilization: Ensures the availability of energy resources during heightened activity levels.
- Redirecting Blood Flow: Optimizes blood distribution to vital organs like the heart and muscles while reducing flow to less critical systems.
Parasympathetic Nervous System (PNS):
Mechanism of Action:
- Neurotransmitters: Primarily uses acetylcholine as its main neurotransmitter at target organs.
- Receptors: Acts on muscarinic and nicotinic acetylcholine receptors located on effector organs.
- Ganglia: Consists of paired cranial nerves (e.g., vagus nerve) and sacral spinal nerves, with preganglionic neurons releasing acetylcholine to stimulate postganglionic neurons.
Physiological Effects:
- Rest and Digest Response: Promotes relaxation and conservation of energy by enhancing bodily functions.
- Cardiovascular System: Decreases heart rate and contractility.
- Respiratory System: Constricts bronchioles to reduce airflow when not needed.
- Metabolic Effects: Promotes glycogen synthesis and lipogenesis, storing energy for future use.
- Digestive System: Stimulates digestive activities, increasing peristalsis and enzyme secretion.
- Pupillary Response: Causes pupil constriction (miosis) to protect the eyes from excessive light.
Role in Homeostasis:
- Energy Conservation: Ensures the body conserves energy during periods of rest and recovery.
- Maintenance of Bodily Functions: Supports ongoing physiological processes like digestion and nutrient absorption.
- Balancing SNS Activity: Counteracts the sympathetic nervous system to maintain internal balance and prevent overstimulation.
Balancing Mechanisms:
Functional Complementarity:
- The SNS and PNS often have opposing effects on the same organs, allowing for fine-tuned regulation of physiological processes.
- Example: While the SNS increases heart rate, the PNS decreases it, enabling the body to rapidly adjust to changing demands.
Feedback Systems:
- Negative feedback loops ensure that physiological parameters remain within optimal ranges by adjusting SNS and PNS activity as needed.
- Example: After a stressful event, PNS activity increases to restore heart rate and blood pressure to baseline levels.
Integration with Other Systems:
- The ANS works in conjunction with the endocrine and immune systems to coordinate comprehensive responses to internal and external stimuli.
Clinical Relevance:
Autonomic Dysregulation:
- Conditions: Disorders like dysautonomia, where there is improper functioning of the ANS, can lead to symptoms such as abnormal heart rates, blood pressure fluctuations, and digestive issues.
Pharmacological Interventions:
- Sympathomimetics: Drugs that mimic SNS activity (e.g., epinephrine) are used to treat conditions like asthma and cardiac arrest.
- Parasympathomimetics: Drugs that mimic PNS activity (e.g., pilocarpine) are used to treat glaucoma and dry mouth.
Stress Management:
- Techniques that enhance PNS activity, such as meditation and deep breathing, can help counteract the negative effects of chronic stress by promoting relaxation and reducing SNS overactivity.
Conclusion: The sympathetic and parasympathetic divisions of the autonomic nervous system work in tandem to maintain homeostasis by balancing the body’s readiness for action with its need for rest and recovery. Through their opposing yet complementary actions, they regulate vital physiological processes, ensuring that the body responds appropriately to various internal and external challenges. Understanding the mechanisms and functions of these divisions is essential for diagnosing and managing autonomic-related disorders and for developing strategies to enhance overall health and well-being.
Question 7 (Digestion)
Outline the process of digestion, highlighting the roles of the major digestive organs and the key enzymatic actions involved in nutrient breakdown.
Answer:
Digestion Process Overview: Digestion is the biological process through which the body breaks down food into smaller molecules that can be absorbed and utilized for energy, growth, and repair. It involves both mechanical and chemical processes carried out by various digestive organs and enzymes.
Major Digestive Organs and Their Roles:
Mouth:
Functions:
- Mechanical Digestion: Chewing (mastication) breaks food into smaller pieces, increasing the surface area for enzymatic action.
- Chemical Digestion: Salivary glands secrete saliva containing the enzyme amylase, which begins the breakdown of carbohydrates into simpler sugars.
- Formation of Bolus: The tongue helps mix food with saliva and forms it into a manageable mass (bolus) for swallowing.
Pharynx and Esophagus:
Functions:
- Transport: The pharynx serves as a pathway for food from the mouth to the esophagus.
- Peristalsis: Coordinated, wave-like muscle contractions in the esophagus propel the bolus toward the stomach.
Stomach:
Functions:
- Mechanical Digestion: Churns food to mix it with gastric juices, further breaking it down into a semi-liquid mixture called chyme.
- Chemical Digestion: Secretes hydrochloric acid (HCl) and the enzyme pepsin, initiating protein breakdown into peptides.
- Protection: The acidic environment kills most ingested pathogens.
- Storage: Temporarily holds food, releasing chyme into the small intestine in controlled amounts.
Small Intestine:
Structure: Comprises three parts—duodenum, jejunum, and ileum.
Functions:
- Chemical Digestion: Receives digestive enzymes from the pancreas and bile from the liver to continue breaking down carbohydrates, proteins, and fats.
- Duodenum: Primary site for enzymatic digestion; pancreatic amylase breaks down carbohydrates, trypsin and chymotrypsin digest proteins, and lipase breaks down fats.
- Bile: Produced by the liver and stored in the gallbladder; emulsifies fats, increasing their surface area for lipase action.
- Nutrient Absorption: Villi and microvilli in the jejunum and ileum increase surface area for efficient absorption of nutrients into the bloodstream.
- Hormonal Regulation: Secretes hormones like secretin and cholecystokinin (CCK) that regulate enzyme and bile secretion.
- Chemical Digestion: Receives digestive enzymes from the pancreas and bile from the liver to continue breaking down carbohydrates, proteins, and fats.
Pancreas:
Functions:
- Enzyme Secretion: Produces digestive enzymes (amylase, lipase, proteases) released into the small intestine to aid in the breakdown of carbohydrates, fats, and proteins.
- Hormone Secretion: Releases insulin and glucagon into the bloodstream to regulate blood glucose levels.
Liver and Gallbladder:
Liver Functions:
- Bile Production: Synthesizes bile, which is essential for fat emulsification.
- Metabolism: Processes nutrients absorbed from the small intestine.
Gallbladder Functions:
- Bile Storage: Concentrates and stores bile, releasing it into the small intestine during digestion.
Large Intestine (Colon):
Functions:
- Water Absorption: Reabsorbs water and electrolytes from undigested food, forming solid waste (feces).
- Bacterial Fermentation: Houses beneficial bacteria that ferment undigested carbohydrates, producing short-chain fatty acids and vitamins.
- Storage and Elimination: Stores feces until they are expelled through defecation.
Rectum and Anus:
Functions:
- Storage: The rectum temporarily holds feces.
- Excretion: The anus controls the release of feces from the body through voluntary and involuntary muscle contractions.
Key Enzymatic Actions:
- Amylase:
- Location: Salivary glands (salivary amylase) and pancreas (pancreatic amylase).
- Function: Breaks down starches into maltose and dextrins.
- Pepsin:
- Location: Stomach.
- Function: Initiates protein digestion by breaking peptide bonds, producing smaller peptides.
- Trypsin and Chymotrypsin:
- Location: Small intestine (from the pancreas).
- Function: Further break down peptides into amino acids and smaller peptides.
- Lipase:
- Location: Pancreas.
- Function: Breaks down triglycerides into free fatty acids and monoglycerides.
- Lactase, Sucrase, and Maltase:
- Location: Small intestine (brush border enzymes).
- Function: Hydrolyze disaccharides (lactose, sucrose, maltose) into monosaccharides (glucose, fructose, galactose) for absorption.
Regulation of Digestion:
- Neural Control:
- Enteric Nervous System: Independent network that coordinates digestive processes.
- Autonomic Nervous System: SNS stimulates digestive inhibition, while PNS promotes digestive activities.
- Hormonal Control:
- Gastrin: Stimulates acid secretion in the stomach.
- Secretin: Promotes bicarbonate secretion from the pancreas to neutralize stomach acid.
- Cholecystokinin (CCK): Stimulates enzyme and bile release, slows gastric emptying.
Clinical Relevance:
- Digestive Disorders:
- Gastritis: Inflammation of the stomach lining affecting acid and enzyme secretion.
- Lactose Intolerance: Deficiency of lactase leading to impaired lactose digestion.
- Celiac Disease: Immune reaction to gluten affecting nutrient absorption in the small intestine.
- Enzyme Deficiencies:
- Pancreatic Insufficiency: Reduced secretion of digestive enzymes, leading to malabsorption and nutrient deficiencies.
- Obstructive Conditions:
- Gallstones: Blockage of bile ducts, impairing fat digestion and absorption.
Conclusion: The digestive process is a highly coordinated series of mechanical and chemical actions involving multiple organs and enzymes. From the initial breakdown of food in the mouth to the absorption of nutrients in the small intestine and the elimination of waste in the large intestine, each component plays a specific role in ensuring efficient nutrient utilization and waste removal. Understanding the structure and function of the digestive system is essential for diagnosing and managing various gastrointestinal disorders, promoting overall health and well-being.
Question 8 (Respiratory System)
Describe the structure and function of the respiratory system, focusing on the mechanics of breathing and gas exchange in the lungs.
Answer:
Respiratory System Overview: The respiratory system is responsible for the intake of oxygen (O₂) and the expulsion of carbon dioxide (CO₂), a metabolic waste product. It consists of the upper and lower respiratory tracts, culminating in the lungs, where gas exchange occurs.
Structure of the Respiratory System:
Upper Respiratory Tract:
- Nose and Nasal Cavity:
- Function: Filters, warms, and humidifies incoming air; olfactory receptors detect smells.
- Structure: Lined with mucous membranes and cilia that trap debris and pathogens.
- Pharynx (Throat):
- Function: Serves as a passageway for air (to the larynx) and food (to the esophagus).
- Structure: Divided into nasopharynx, oropharynx, and laryngopharynx.
- Larynx (Voice Box):
- Function: Routes air into the lower respiratory tract and protects the trachea against food aspiration; houses vocal cords for sound production.
- Structure: Composed of cartilaginous rings and muscles that control pitch and volume of voice.
- Nose and Nasal Cavity:
Lower Respiratory Tract:
- Trachea (Windpipe):
- Function: Conducts air from the larynx to the bronchi.
- Structure: Reinforced with C-shaped cartilaginous rings to maintain an open airway.
- Bronchi and Bronchioles:
- Function: Distribute air to the lungs and further branch into smaller airways.
- Structure: Bronchi branch into smaller bronchioles, which lack cartilage and lead to the alveolar ducts.
- Alveoli:
- Function: Site of gas exchange between air and blood.
- Structure: Tiny, balloon-like sacs with thin walls and a large surface area; surrounded by capillaries.
- Lungs:
- Function: Contain the alveoli and facilitate the exchange of gases.
- Structure: Divided into lobes (three in the right lung, two in the left lung); enveloped by a double-layered pleural membrane.
- Trachea (Windpipe):
Mechanics of Breathing:
Inhalation (Inspiration):
- Diaphragm and Intercostal Muscles: The diaphragm contracts and flattens, while the external intercostal muscles contract, expanding the thoracic cavity.
- Pressure Changes: Expansion increases thoracic volume, creating negative pressure relative to atmospheric pressure, causing air to flow into the lungs.
- Air Flow: Air moves from high-pressure areas (atmosphere) to low-pressure areas (lungs) until equilibrium is reached.
Exhalation (Expiration):
- Passive Process: Diaphragm and intercostal muscles relax, reducing thoracic volume and increasing pressure within the lungs.
- Pressure Changes: Positive pressure forces air out of the lungs into the atmosphere.
- Air Flow: Air flows from areas of higher pressure (lungs) to lower pressure (atmosphere).
Gas Exchange in the Lungs:
Alveolar-Capillary Interface:
- Structure: Alveoli are surrounded by a dense network of capillaries, creating a thin barrier (respiratory membrane) for efficient gas diffusion.
- Components: Comprises alveolar epithelium, capillary endothelium, and a shared basement membrane.
Diffusion of Gases:
Oxygen Uptake: O₂ diffuses from the alveolar air (high pO₂) into the blood (low pO₂) across the respiratory membrane.
Carbon Dioxide Removal: CO₂ diffuses from the blood (high pCO₂) into the alveolar air (low pCO₂) to be exhaled.
Factors Affecting Diffusion:
- Partial Pressure Gradient: The difference in pO₂ and pCO₂ between alveoli and blood drives diffusion.
- Surface Area: Large surface area of alveoli enhances gas exchange efficiency.
- Membrane Thickness: Thin respiratory membrane facilitates rapid gas diffusion.
- Solubility of Gases: CO₂ is more soluble in blood than O₂, allowing it to diffuse more readily.
Hemoglobin’s Role in Oxygen Transport:
- Binding O₂: Hemoglobin molecules in red blood cells bind oxygen in the lungs, enhancing O₂ transport capacity.
- Release O₂: In tissues, hemoglobin releases O₂ due to lower pO₂, ensuring efficient delivery to cells.
Regulation of Breathing:
Medulla Oblongata:
- Respiratory Centers: Control the rate and depth of breathing through neural signals to respiratory muscles.
Chemoreceptors:
Peripheral Chemoreceptors: Located in the carotid and aortic bodies, detect changes in blood pH, pCO₂, and pO₂.
Central Chemoreceptors: Located in the medulla, primarily sensitive to changes in cerebrospinal fluid pH due to CO₂ levels.
Response to CO₂ Levels: Elevated CO₂ (lower pH) stimulates increased breathing rate and depth to expel excess CO₂.
Mechanoreceptors:
- Stretch Receptors: Located in the lungs and airways, detect lung inflation and signal to reduce breathing rate when lungs are full (Hering-Breuer reflex).
Clinical Relevance:
Respiratory Disorders:
- Asthma: Involves bronchoconstriction, reducing airflow and impairing gas exchange.
- Chronic Obstructive Pulmonary Disease (COPD): Characterized by obstructed airflow and impaired gas exchange.
- Pulmonary Fibrosis: Thickening and scarring of alveolar walls hinder gas diffusion.
Ventilation Issues:
- Hyperventilation: Excessive breathing leading to reduced blood CO₂ levels and respiratory alkalosis.
- Hypoventilation: Insufficient breathing resulting in elevated blood CO₂ levels and respiratory acidosis.
Medical Interventions:
- Mechanical Ventilation: Supports or replaces natural breathing in patients with respiratory failure.
- Oxygen Therapy: Provides supplemental oxygen to enhance O₂ delivery in conditions like pneumonia or heart failure.
Conclusion: The respiratory system is integral to maintaining the body’s oxygen supply and carbon dioxide removal, ensuring cellular respiration and metabolic functions. Through the coordinated actions of the heart, lungs, and respiratory muscles, along with precise regulation by neural and chemical feedback mechanisms, the system maintains efficient gas exchange and overall homeostasis. Understanding the structure and function of the respiratory system is essential for diagnosing and treating various pulmonary disorders, promoting effective interventions to support respiratory health.
Question 9 (Kidney)
Explain the role of the kidneys in maintaining electrolyte balance and blood pressure, detailing the mechanisms involved in these processes.
Answer:
Kidneys Overview: The kidneys are vital organs responsible for filtering blood, removing waste products, regulating fluid and electrolyte balance, and maintaining overall homeostasis, including blood pressure regulation. Each kidney contains approximately one million nephrons, the functional units that carry out these essential processes.
Maintaining Electrolyte Balance:
Filtration and Reabsorption:
- Glomerular Filtration: Blood enters the nephron through the glomerulus, where water and solutes are filtered into the Bowman’s capsule, forming filtrate.
- Proximal Tubule Reabsorption: Essential electrolytes like sodium (Na⁺), potassium (K⁺), calcium (Ca²⁺), and chloride (Cl⁻) are reabsorbed from the filtrate back into the bloodstream through active and passive transport mechanisms.
Selective Reabsorption and Secretion:
- Loop of Henle: Concentrates urine by creating a hyperosmotic medullary interstitium, facilitating the reabsorption of Na⁺ and Cl⁻.
- Distal Tubule and Collecting Duct:
- Regulated Reabsorption: Hormones like aldosterone increase Na⁺ reabsorption and K⁺ secretion to maintain electrolyte balance.
- Calcium Regulation: Parathyroid hormone (PTH) regulates Ca²⁺ reabsorption based on the body’s needs.
Excretion:
- Waste Removal: Excess electrolytes that are not reabsorbed are excreted in the urine, preventing imbalances.
- Regulatory Hormones: Hormones like antidiuretic hormone (ADH) influence water reabsorption, indirectly affecting electrolyte concentrations.
Maintaining Blood Pressure:
Renin-Angiotensin-Aldosterone System (RAAS):
- Renin Release: Low blood pressure or low Na⁺ levels trigger the juxtaglomerular cells in the kidneys to release renin.
- Angiotensin II Formation: Renin converts angiotensinogen (produced by the liver) to angiotensin I, which is then converted to angiotensin II by angiotensin-converting enzyme (ACE) in the lungs.
- Effects of Angiotensin II:
- Vasoconstriction: Narrows blood vessels, increasing vascular resistance and blood pressure.
- Aldosterone Secretion: Stimulates the adrenal cortex to release aldosterone, promoting Na⁺ and water reabsorption, increasing blood volume and pressure.
- ADH Release: Promotes water retention by the kidneys, further increasing blood volume.
Natriuretic Peptides:
- Atrial Natriuretic Peptide (ANP): Released by atrial cells in response to high blood volume, ANP promotes Na⁺ excretion, reducing blood volume and pressure.
Kidney Function in Blood Volume Regulation:
- Fluid Balance: By regulating the excretion or retention of water and electrolytes, kidneys control blood volume, directly influencing blood pressure.
Autoregulation:
- Renal Arterial Autoregulation: Maintains consistent blood flow to the kidneys despite fluctuations in systemic blood pressure, ensuring stable glomerular filtration rates.
Mechanisms Involved:
- Filtration Rate Regulation:
- Glomerular Filtration Rate (GFR): Controlled by afferent and efferent arteriole constriction, adjusting blood flow and pressure within the glomerulus.
- Tubuloglomerular Feedback:
- Macula Densa Role: Senses Na⁺Cl⁻ concentration in the distal tubule and adjusts afferent arteriole tone accordingly to regulate GFR.
- Sympathetic Nervous System:
- Impact on RAAS: Activates renin release and vasoconstriction, contributing to increased blood pressure during stress or blood loss.
Clinical Relevance:
- Hypertension:
- Role of RAAS: Overactivation of the RAAS can lead to sustained vasoconstriction and increased blood volume, contributing to high blood pressure.
- Therapeutic Targets: ACE inhibitors and angiotensin II receptor blockers (ARBs) are used to manage hypertension by disrupting the RAAS pathway.
- Electrolyte Imbalances:
- Conditions: Disorders like hyperkalemia (high K⁺) or hypokalemia (low K⁺) result from improper regulation of electrolyte reabsorption and secretion.
- Management: Adjusting diuretic therapy or hormone levels can restore balance.
- Chronic Kidney Disease (CKD):
- Impact: Impaired kidney function disrupts electrolyte balance and blood pressure regulation, necessitating interventions like dialysis or transplantation.
Conclusion: The kidneys play a pivotal role in maintaining electrolyte balance and regulating blood pressure, essential components of bodily homeostasis. Through intricate mechanisms involving filtration, selective reabsorption, hormonal control, and interaction with the renin-angiotensin-aldosterone system, kidneys ensure the stability of internal conditions despite external fluctuations. Understanding these processes is crucial for diagnosing and treating conditions related to electrolyte imbalances and hypertension, highlighting the kidneys’ central role in overall health and physiological regulation.
Question 10 (Blood Clotting or Blood Coagulation)
Describe the process of blood clotting (coagulation), including the roles of platelets and the coagulation cascade.
Answer:
Blood Clotting (Coagulation) Overview: Blood clotting is a vital physiological process that prevents excessive bleeding when blood vessels are injured. It involves a complex series of events that culminate in the formation of a stable clot, sealing the wound and facilitating tissue repair. The process is orchestrated by platelets and a cascade of coagulation factors—proteins that activate one another in a stepwise manner.
Key Components Involved:
Platelets (Thrombocytes):
- Role: Act as the first responders to vascular injury, initiating clot formation.
- Structure: Small, anucleate cell fragments derived from megakaryocytes in the bone marrow.
- Function: Adhere to exposed collagen and other subendothelial structures at the injury site, aggregate to form a platelet plug, and release granules containing clotting factors and signaling molecules.
Coagulation Factors:
- Nature: A series of plasma proteins, most of which are serine proteases, that interact in a cascade to form a fibrin clot.
- Number: Traditionally labeled as Factors I (fibrinogen) through XIII, each with specific roles in the clotting process.
Phases of Blood Clotting:
Vascular Phase:
- Vasoconstriction: Immediate constriction of the injured blood vessel reduces blood flow and blood loss.
- Platelet Adhesion: Platelets adhere to exposed collagen and other matrix proteins at the injury site through receptors like glycoprotein Ia/IIa and Ib/IX/V.
Platelet Phase:
- Platelet Activation: Adhered platelets become activated, changing shape to extend pseudopodia for better adhesion and aggregation.
- Platelet Plug Formation: Activated platelets release granules containing ADP, serotonin, and thromboxane A₂, which recruit and activate additional platelets, forming a temporary platelet plug.
- Positive Feedback: Released substances amplify platelet activation and aggregation.
Coagulation Phase:
Coagulation Cascade: A sequential activation of coagulation factors leading to the conversion of fibrinogen to fibrin.
Intrinsic and Extrinsic Pathways:
- Intrinsic Pathway: Triggered by damage within the blood vessel, involving factors XII, XI, IX, and VIII.
- Extrinsic Pathway: Initiated by external trauma, releasing tissue factor (Factor III) which interacts with Factor VII.
- Common Pathway: Both pathways converge on Factor X, leading to the conversion of prothrombin (Factor II) to thrombin (Factor IIa).
Thrombin Formation: Thrombin plays a central role by converting fibrinogen into fibrin monomers.
Fibrin Clot Formation: Fibrin monomers polymerize to form a stable, insoluble fibrin mesh that reinforces the platelet plug.
Factor XIII Activation: Thrombin activates Factor XIII, which cross-links fibrin strands, stabilizing the clot.
Clot Retraction and Repair:
- Clot Retraction: Platelets contract, pulling the fibrin mesh tighter, reducing the size of the wound and facilitating tissue repair.
Fibrinolysis (Clot Removal):
- Plasmin Activation: Once the vessel is repaired, plasminogen is converted to plasmin, which digests fibrin and dissolves the clot.
- Regulation: Inhibitors like alpha-2 antiplasmin prevent excessive fibrinolysis, maintaining clot stability until healing is complete.
Regulation of Clotting:
Anticoagulants:
- Natural Anticoagulants: Include proteins like antithrombin III, protein C, and protein S that inhibit various steps in the coagulation cascade.
- Fibrinolytic Inhibitors: Prevent premature clot breakdown by inhibiting plasmin activity.
Endothelial Cell Functions:
- Secretion of Inhibitors: Healthy endothelial cells release substances like prostacyclin and nitric oxide that inhibit platelet aggregation and vasoconstriction.
- Tissue Factor Pathway Inhibitor (TFPI): Prevents excessive activation of the extrinsic pathway.
Clinical Relevance:
- Bleeding Disorders:
- Hemophilia: Deficiency in specific coagulation factors (e.g., Factor VIII in Hemophilia A) impairs clot formation, leading to excessive bleeding.
- Thrombosis:
- Formation of Pathological Clots: Excessive clotting can result in conditions like deep vein thrombosis (DVT) or pulmonary embolism, obstructing blood flow.
- Anticoagulant Therapies:
- Warfarin and Heparin: Medications that inhibit various steps in the coagulation cascade to prevent unwanted clot formation in patients at risk of thrombosis.
- Clotting Factor Concentrates:
- Therapeutic Use: Administered to individuals with clotting factor deficiencies to restore normal clotting function.
Conclusion: Blood clotting is a meticulously regulated process involving platelets and a cascade of coagulation factors to form a stable clot that prevents excessive bleeding and facilitates wound healing. Understanding the intricate steps and regulatory mechanisms of coagulation is essential for diagnosing and managing various bleeding and clotting disorders. Advances in medical interventions targeting specific components of the clotting process have significantly improved outcomes for patients with coagulation-related conditions.
Question 11 (Endocrine System)
Outline the mechanisms by which the endocrine system regulates metabolism, including the roles of key hormones like insulin and glucagon.
Answer:
Endocrine System Overview: The endocrine system comprises glands that secrete hormones directly into the bloodstream, enabling long-distance communication to regulate various physiological processes, including metabolism, growth, reproduction, and homeostasis. Key endocrine glands involved in metabolic regulation include the pancreas, thyroid, adrenal glands, and pituitary gland.
Regulation of Metabolism: Metabolism encompasses all biochemical reactions that occur within the body, including those that convert food into energy and build or repair tissues. The endocrine system modulates metabolic processes primarily through hormone secretion.
Key Hormones Regulating Metabolism:
Insulin:
Produced By:
- Pancreas: Specifically by the beta cells of the islets of Langerhans.
Functions:
- Glucose Uptake: Facilitates the uptake of glucose into cells, particularly in muscle and adipose tissue, by promoting the translocation of glucose transporter type 4 (GLUT4) to the cell membrane.
- Glycogenesis: Stimulates the conversion of glucose to glycogen for storage in the liver and muscles.
- Lipogenesis: Promotes the synthesis of fatty acids and triglycerides in adipose tissue.
- Protein Synthesis: Enhances amino acid uptake and protein synthesis in cells.
Effects on Blood Glucose Levels:
- Lowering Blood Glucose: Insulin decreases blood glucose levels by promoting cellular uptake and storage.
Glucagon:
Produced By:
- Pancreas: Specifically by the alpha cells of the islets of Langerhans.
Functions:
- Glycogenolysis: Stimulates the breakdown of glycogen to glucose in the liver, releasing glucose into the bloodstream.
- Gluconeogenesis: Promotes the synthesis of glucose from non-carbohydrate sources in the liver.
- Lipolysis: Enhances the breakdown of triglycerides into free fatty acids and glycerol in adipose tissue.
Effects on Blood Glucose Levels:
- Raising Blood Glucose: Glucagon increases blood glucose levels by promoting glycogen breakdown and glucose production.
Thyroid Hormones (T3 and T4):
Produced By:
- Thyroid Gland: Follicular cells synthesize triiodothyronine (T3) and thyroxine (T4).
Functions:
- Basal Metabolic Rate (BMR): Increase BMR by enhancing oxygen consumption and heat production in cells.
- Protein Synthesis and Degradation: Regulate the rate of protein turnover in tissues.
- Carbohydrate Metabolism: Promote glycolysis and glycogenolysis.
- Lipid Metabolism: Enhance lipolysis and cholesterol synthesis.
Effects on Metabolism:
- Metabolic Acceleration: Thyroid hormones elevate overall metabolic activity, affecting multiple organ systems.
Adrenaline (Epinephrine) and Noradrenaline (Norepinephrine):
Produced By:
- Adrenal Medulla: Part of the adrenal glands.
Functions:
- Glycogenolysis and Gluconeogenesis: Stimulate the release of glucose from liver and muscles.
- Lipolysis: Promote the breakdown of fats into free fatty acids.
- Increased Heart Rate and Blood Pressure: Enhance blood flow to muscles and vital organs.
- Energy Mobilization: Prepare the body for ‘fight or flight’ responses by increasing available energy substrates.
Effects on Metabolism:
- Immediate Energy Supply: Rapidly increases blood glucose and fatty acid levels to meet acute energy demands.
Mechanisms of Hormonal Regulation:
Feedback Loops:
- Negative Feedback: The primary mechanism for maintaining hormonal balance. For example, high blood glucose levels stimulate insulin release, which lowers glucose levels, subsequently reducing insulin secretion.
- Positive Feedback: Less common, but occurs in processes like childbirth, where oxytocin release increases contractions, further stimulating more oxytocin release.
Receptor Interaction:
- Cell Surface Receptors: Many metabolic hormones (e.g., insulin, glucagon) bind to receptors on the cell membrane, initiating signal transduction pathways that alter cellular metabolism.
- Intracellular Receptors: Some hormones (e.g., thyroid hormones) pass through the cell membrane and bind to receptors within the cell, directly influencing gene expression and protein synthesis.
Hormone Synthesis and Secretion Regulation:
- Hypothalamic Control: The hypothalamus regulates the secretion of pituitary hormones, which in turn control other endocrine glands.
- Autocrine and Paracrine Signals: Some hormones act on the cells that produce them (autocrine) or on nearby cells (paracrine), providing localized regulation of metabolic processes.
Clinical Relevance:
Diabetes Mellitus:
- Type 1 Diabetes: Autoimmune destruction of insulin-producing beta cells, leading to insulin deficiency and hyperglycemia.
- Type 2 Diabetes: Insulin resistance and relative insulin deficiency, resulting in impaired glucose uptake and elevated blood glucose levels.
- Management: Involves insulin therapy, oral hypoglycemic agents, and lifestyle modifications to regulate blood glucose.
Hypothyroidism and Hyperthyroidism:
- Hypothyroidism: Insufficient thyroid hormone production, causing reduced metabolic rate, weight gain, and fatigue.
- Hyperthyroidism: Excessive thyroid hormone production, leading to increased metabolic rate, weight loss, and anxiety.
- Treatment: Hormone replacement for hypothyroidism and antithyroid medications or surgery for hyperthyroidism.
Adrenal Disorders:
- Addison’s Disease: Insufficient production of adrenal hormones (e.g., cortisol, aldosterone), leading to hypotension, electrolyte imbalances, and fatigue.
- Cushing’s Syndrome: Excessive cortisol production, causing weight gain, hypertension, and hyperglycemia.
- Treatment: Hormone replacement therapy for Addison’s and surgical or medical interventions to reduce cortisol levels in Cushing’s.
Conclusion: The endocrine system intricately regulates metabolism through a network of hormones that control nutrient uptake, energy storage, and energy mobilization. Hormones like insulin and glucagon play pivotal roles in maintaining blood glucose levels, while thyroid hormones and catecholamines modulate overall metabolic rate and energy availability. Understanding these regulatory mechanisms is essential for diagnosing and managing metabolic disorders, ensuring that the body’s energy needs are met efficiently and effectively.
Question 12 (Kidney Regulation and Kidney Dysregulation)
How do the kidneys regulate acid-base balance in the body, and what are the consequences of dysregulation in this system?
Answer:
Acid-Base Balance Overview: Maintaining the body’s acid-base balance is crucial for normal cellular function and overall homeostasis. The pH of blood and extracellular fluid must be tightly regulated within a narrow range (approximately 7.35 to 7.45). The kidneys play a pivotal role in this regulation by controlling the excretion and reabsorption of hydrogen ions (H⁺) and bicarbonate ions (HCO₃⁻).
Kidneys’ Role in Acid-Base Regulation:
Bicarbonate Reabsorption:
- Proximal Tubule Function: Approximately 85-90% of filtered bicarbonate is reabsorbed in the proximal tubule.
- Mechanism:
- Carbonic Anhydrase Activity: Inside tubular cells, carbonic anhydrase catalyzes the conversion of CO₂ and H₂O to carbonic acid (H₂CO₃), which dissociates into H⁺ and HCO₃⁻.
- H⁺ Secretion: H⁺ ions are secreted into the tubular lumen via Na⁺/H⁺ exchangers, while HCO₃⁻ is transported back into the blood through anion exchangers.
- Buffering: In the lumen, H⁺ ions combine with filtered HCO₃⁻ and other buffer systems (e.g., ammonia) to form bicarbonate, which prevents acidification of the tubular fluid.
Hydrogen Ion Secretion:
- Distal Tubule and Collecting Duct Function: Actively secretes additional H⁺ ions into the tubular fluid, especially in response to metabolic acidosis.
- Mechanism:
- Active Transport: H⁺ ions are pumped into the lumen via H⁺-ATPase and H⁺/K⁺-ATPase pumps.
- Ammoniagenesis: The kidneys produce ammonia (NH₃) from glutamine metabolism, which buffers H⁺ ions by forming ammonium (NH₄⁺) for excretion.
Phosphate Buffer System:
- Role: Bicarbonate is reabsorbed in exchange for phosphate ions, which bind H⁺ ions and are excreted in the urine.
Generation of New Bicarbonate:
- Buffering and Metabolic Reactions: The kidneys generate new bicarbonate ions by secreting H⁺ and combining it with filtered bicarbonate and other buffers.
Regulatory Hormones and Mechanisms:
Aldosterone:
- Function: Promotes Na⁺ reabsorption and K⁺ secretion in the distal tubule and collecting ducts, indirectly enhancing H⁺ secretion to maintain electroneutrality.
Antidiuretic Hormone (ADH):
- Function: Regulates water reabsorption, indirectly influencing blood osmolarity and acid-base balance by diluting or concentrating the blood.
Renin-Angiotensin-Aldosterone System (RAAS):
- Role: In response to low blood pressure or low Na⁺ levels, RAAS activation leads to increased aldosterone secretion, enhancing H⁺ and Na⁺ reabsorption.
Consequences of Dysregulation:
Metabolic Acidosis:
- Definition: A condition where the body produces excessive H⁺ ions or loses too much bicarbonate.
- Kidney Response: Increases H⁺ secretion and bicarbonate reabsorption, along with enhanced ammoniagenesis and phosphate buffering.
- Symptoms: Rapid breathing, confusion, fatigue, and in severe cases, shock or coma.
Metabolic Alkalosis:
- Definition: A condition where the body loses excessive H⁺ ions or gains too much bicarbonate.
- Kidney Response: Decreases H⁺ secretion and may increase bicarbonate excretion, while conserving H⁺ ions.
- Symptoms: Muscle twitching, hand tremors, nausea, vomiting, and prolonged muscle spasms.
Respiratory Acidosis:
- Cause: Impaired ventilation leading to CO₂ retention and increased H⁺ concentration.
- Kidney Compensation: Enhances bicarbonate reabsorption and H⁺ secretion to buffer the excess acidity.
Respiratory Alkalosis:
- Cause: Hyperventilation causing excessive CO₂ loss and decreased H⁺ concentration.
- Kidney Compensation: Reduces bicarbonate reabsorption and increases H⁺ excretion to restore balance.
Clinical Relevance:
- Chronic Kidney Disease (CKD):
- Impact: Impaired kidney function reduces the ability to excrete H⁺ and reabsorb HCO₃⁻, leading to metabolic acidosis.
- Management: May require bicarbonate supplementation and dietary modifications to restore acid-base balance.
- Diabetic Ketoacidosis (DKA):
- Mechanism: Excessive ketone production (acids) overwhelms the buffering capacity, causing metabolic acidosis.
- Kidney Role: Attempts to compensate by increasing bicarbonate generation and H⁺ excretion.
- Vomiting and Diuretic Use:
- Effect: Loss of stomach acid (vomiting) or excessive diuresis can lead to metabolic alkalosis.
- Kidney Response: Adjusts bicarbonate handling to mitigate alkalosis.
Conclusion: The kidneys are central to maintaining the body’s acid-base balance through intricate mechanisms involving the regulation of hydrogen and bicarbonate ions. By adjusting secretion, reabsorption, and generation of buffers, the kidneys respond dynamically to shifts in pH, ensuring that physiological processes remain within optimal ranges. Dysregulation of these mechanisms can lead to serious acid-base disturbances, highlighting the critical role of renal function in overall homeostasis and health.
Question 13 (Endocrine-Related Genetic Disorders)
[Extra question beyond the requested 12] How do genetic factors influence the development and function of the endocrine system, and what are the implications of endocrine-related genetic disorders?
Answer:
Genetic Influence on the Endocrine System:
Development of Endocrine Glands:
- Genetic Regulation: The formation and differentiation of endocrine glands (e.g., pituitary, thyroid, adrenal glands) are governed by specific genes and transcription factors.
- Gene Expression: Proper expression of genes like PAX6 (eye and pancreas development) and Hox genes (regional identity) is crucial for the correct anatomical and functional development of endocrine structures.
Hormone Production and Secretion:
- Gene Coding: Genes encode the proteins and enzymes necessary for synthesizing hormones. Mutations can affect hormone production, leading to deficiencies or excesses.
- Signal Transduction: Genetic variations in hormone receptors or intracellular signaling molecules can alter the responsiveness of target cells to endocrine signals.
Regulation and Feedback Mechanisms:
- Negative Feedback Loops: Genes involved in feedback pathways ensure that hormone levels remain within physiological ranges. Genetic disruptions can impair these regulatory mechanisms.
- Receptor Sensitivity: Variations in receptor genes can affect how effectively hormones bind and trigger cellular responses, influencing overall endocrine function.
Endocrine-Related Genetic Disorders:
Congenital Hypothyroidism:
- Cause: Genetic mutations affecting thyroid hormone synthesis (e.g., TPO gene mutations) or thyroid gland development (e.g., NKX2-1 mutations).
- Implications: If untreated, can lead to intellectual disability, growth retardation, and metabolic issues. Early diagnosis and hormone replacement therapy are essential.
Multiple Endocrine Neoplasia (MEN) Syndromes:
- Types: MEN1, MEN2A, and MEN2B, each associated with mutations in different genes (e.g., MEN1 gene for MEN1).
- Implications: Characterized by tumors in multiple endocrine glands, such as parathyroid, pituitary, and adrenal glands (MEN1), or thyroid and adrenal glands (MEN2). Requires vigilant monitoring and management to prevent malignancies.
Addison’s Disease:
- Cause: Often autoimmune, but can result from genetic defects in steroidogenesis enzymes (e.g., CYP21A2 gene).
- Implications: Leads to insufficient production of cortisol and aldosterone, causing fatigue, muscle weakness, hypotension, and electrolyte imbalances. Managed with hormone replacement therapy.
Cushing’s Syndrome:
- Cause: Genetic mutations can lead to adrenal adenomas producing excessive cortisol (e.g., PRKACA gene mutations).
- Implications: Results in weight gain, hypertension, osteoporosis, and hyperglycemia. Treatment involves surgical removal of tumors or medical management to reduce cortisol levels.
Diabetes Mellitus:
- Type 1: Often associated with HLA gene complex mutations, leading to autoimmune destruction of pancreatic beta cells and insulin deficiency.
- Type 2: Involves multiple genes influencing insulin resistance and beta-cell function, alongside environmental factors like obesity.
- Implications: Requires lifelong management with insulin therapy (Type 1) or lifestyle changes and medications to improve insulin sensitivity and secretion (Type 2).
Pituitary Dwarfism:
- Cause: Mutations in growth hormone (GH) genes or factors regulating pituitary development (e.g., PROP1 gene).
- Implications: Characterized by short stature and delayed development, often managed with growth hormone therapy.
Implications of Endocrine-Related Genetic Disorders:
Impact on Growth and Development:
- Hormonal Deficiencies: Can lead to stunted growth, delayed puberty, and developmental delays.
- Hormonal Excesses: May cause overgrowth conditions, precocious puberty, or metabolic syndromes.
Metabolic Dysregulation:
- Energy Balance: Hormones like insulin and thyroid hormones are crucial for metabolism; genetic disorders affecting these hormones can disrupt energy utilization and storage.
Reproductive Health:
- Sex Hormones: Genetic disruptions in hormone production can affect fertility, sexual development, and reproductive functions.
Mental Health and Cognitive Function:
- Neurodevelopmental Effects: Hormones like thyroid hormones are essential for brain development; deficiencies can lead to cognitive impairments and mood disorders.
Cardiovascular Health:
- Blood Pressure Regulation: Hormones like aldosterone and catecholamines influence blood pressure; genetic disorders can lead to hypertension or hypotension.
Management and Treatment:
Genetic Counseling:
- Risk Assessment: Helps families understand the hereditary aspects of endocrine disorders and assess risks for future generations.
Hormone Replacement Therapy:
- Deficiency Conditions: Administering synthetic hormones (e.g., levothyroxine for hypothyroidism) to restore normal physiological function.
Targeted Therapies:
- Tumor Removal: Surgical interventions for endocrine tumors associated with MEN syndromes or other neoplasias.
- Gene Therapy: Emerging approaches aim to correct genetic defects at the molecular level, though still largely experimental.
Lifestyle and Supportive Measures:
- Diet and Exercise: Especially important in managing conditions like Type 2 diabetes.
- Psychological Support: Addressing mental health aspects associated with chronic endocrine disorders.
Conclusion: Genetic factors play a crucial role in the development and function of the endocrine system, influencing hormone synthesis, secretion, and action. Genetic mutations can lead to a variety of endocrine-related disorders, each with distinct clinical manifestations and implications for overall health. Understanding the genetic underpinnings of these conditions is essential for accurate diagnosis, effective management, and the development of targeted therapies, ultimately improving patient outcomes and quality of life.
Physiology Thought-Provoking Questions
Based on the information available from the provided URL, here are 12 thought-provoking questions related to physiology, each accompanied by a detailed and elaborate answer:
How does the concept of homeostasis illustrate the body’s ability to maintain internal stability amidst external environmental changes?
Homeostasis refers to the body’s intrinsic ability to maintain a stable internal environment despite fluctuations in the external environment. This dynamic equilibrium is crucial for optimal cellular function and overall survival. For instance, the human body regulates its internal temperature through mechanisms such as sweating to dissipate heat and shivering to generate warmth, ensuring that core temperature remains around 37°C. Similarly, blood glucose levels are tightly controlled by hormones like insulin and glucagon to provide cells with a consistent energy supply. Disruptions in homeostasis can lead to diseases; for example, diabetes mellitus results from the body’s inability to regulate blood glucose levels effectively.
In what ways do the structure and function of cells exemplify the principle of the structure-function relationship in physiology?
The structure-function relationship is a fundamental concept in physiology, emphasizing that the form of a biological structure is inherently linked to its function. At the cellular level, this is evident in various specialized cells. For example, red blood cells have a biconcave shape, increasing their surface area to enhance oxygen absorption and facilitate smooth passage through narrow capillaries. Neurons possess long axons to transmit electrical impulses over distances, while dendrites increase surface area to receive signals from other neurons. The microvilli on intestinal epithelial cells expand surface area, optimizing nutrient absorption. These structural adaptations enable cells to perform their specific functions efficiently.
How do feedback mechanisms contribute to physiological regulation, and what are the differences between negative and positive feedback loops?
Feedback mechanisms are essential for maintaining physiological balance. Negative feedback loops work to counteract deviations from a set point, promoting stability. For instance, when blood pressure rises, baroreceptors in blood vessels detect this change and signal the brain to initiate responses that lower heart rate and dilate blood vessels, reducing blood pressure back to normal levels. Positive feedback loops, in contrast, amplify responses. A classic example is the release of oxytocin during childbirth: uterine contractions stimulate oxytocin release, which intensifies contractions, further increasing oxytocin levels until delivery occurs. While negative feedback maintains homeostasis, positive feedback drives processes to completion.
What role does cell signaling play in coordinating physiological processes across different systems in the body?
Cell signaling is pivotal for communication within and between cells, ensuring coordinated physiological functions. Cells release signaling molecules, such as hormones and neurotransmitters, which bind to specific receptors on target cells, triggering responses. For example, the hormone insulin is secreted by the pancreas in response to elevated blood glucose levels. Insulin binds to receptors on muscle and fat cells, facilitating glucose uptake and lowering blood sugar levels. In the nervous system, neurotransmitters like acetylcholine transmit signals between neurons, enabling rapid responses to stimuli. Disruptions in cell signaling can lead to diseases; for instance, insulin resistance in type 2 diabetes impairs glucose regulation.
How does the cardiovascular system exemplify the integration of multiple physiological systems to maintain homeostasis?
The cardiovascular system integrates with various physiological systems to sustain homeostasis. It transports oxygen, nutrients, hormones, and waste products between tissues and organs. For example, during exercise, muscle activity increases, leading to higher carbon dioxide production and lactic acid accumulation. Chemoreceptors detect these changes, signaling the respiratory system to increase breathing rate, enhancing oxygen intake and carbon dioxide expulsion. Simultaneously, the cardiovascular system elevates heart rate and dilates blood vessels supplying active muscles, ensuring adequate oxygen delivery and waste removal. This coordinated response maintains internal balance during increased physical activity.
In what ways does the study of comparative physiology enhance our understanding of human physiological processes?
Comparative physiology examines the functional characteristics of different organisms to gain insights into human physiology. By studying diverse life forms, scientists can identify universal principles and unique adaptations. For instance, hibernating animals have mechanisms to significantly reduce their metabolic rate, providing clues about metabolic control that could inform medical strategies for managing human metabolic disorders. Research on the regenerative abilities of certain species, like salamanders, offers potential avenues for advancing human tissue regeneration and wound healing. Thus, comparative physiology broadens our understanding of biological functions and their applications to human health.
How do the principles of energy and metabolism apply to the functioning of the human body?
Energy and metabolism are central to physiological processes. The body converts energy from nutrients into adenosine triphosphate (ATP), the primary energy currency used in cellular activities. Metabolic pathways, such as glycolysis, the citric acid cycle, and oxidative phosphorylation, systematically extract and transfer energy. For example, during aerobic respiration, glucose is broken down in the presence of oxygen to produce ATP, water, and carbon dioxide. In anaerobic conditions, like intense exercise, cells can temporarily switch to lactic acid fermentation to generate ATP, though less efficiently. Understanding these pathways is crucial for addressing metabolic disorders and optimizing health.