Ecology
Ecology is the scientific study of interactions among organisms and their environment, encompassing both the biotic and abiotic components of ecosystems. It is a branch of science that links biological principles to environmental issues and is foundational within the broader domain of biology. Understanding these relationships requires insights from multiple subfields, including the cellular-level studies offered by cell biology.
Growth and differentiation, as studied in cell development, shape organisms’ abilities to interact with their surroundings. Processes governing internal conditions, such as those in cell physiology, and the architecture of cell structure influence how organisms adapt to changing environments. The communication between cells ensures that responses to stimuli are coordinated, a feature critical to ecosystem interactions. The cell cycle also plays a role in ecological balance by regulating growth and reproduction.
Ecological studies are enriched by evolutionary perspectives, particularly those grounded in evolutionary biology. Evolution shapes species’ adaptability and survival strategies. These traits are rooted in heredity, which is the domain of genetics and modern fields such as genomics. Patterns observed in Mendelian genetics remain relevant to how traits are distributed across populations and species.
At the molecular level, topics in molecular genetics help explain the mechanisms behind ecological phenomena. The interplay of DNA and RNA, the tools of DNA technology, and the nuances of gene expression are pivotal to understanding how organisms respond to environmental pressures. Disruptions caused by genetic mutations can lead to adaptive advantages or vulnerabilities.
The molecular basis of inheritance offers clues to how characteristics persist in populations, while molecular evolution studies trace how genes have changed across millennia. Advances in molecular techniques in research continue to open new avenues for ecological inquiry, particularly when understanding biodiversity at the genetic level. Similarly, breakthroughs in protein synthesis research inform us about how life processes are maintained across various ecosystems.
Population dynamics—central to ecology—are guided by principles in population genetics, where allele frequencies shift over time due to natural selection, genetic drift, and migration. This intersects with studies in quantitative genetics, which models traits across generations to understand adaptation, survival, and ecological change. Ultimately, ecology acts as a bridge across biological levels of organization, offering students a multidisciplinary lens through which to understand life and sustainability on Earth.
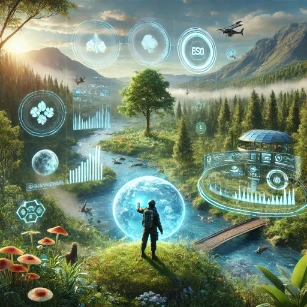
Table of Contents
Key Objectives of Ecology
Understanding Organismal Relationships: Ecology seeks to uncover how organisms interact with one another within populations, communities, and ecosystems. These interactions include competition, predation, mutualism, parasitism, and commensalism.
Exploring Energy Flow and Nutrient Cycling: A major focus of ecology is to understand how energy from the sun is captured, stored, and transferred through food chains and food webs, as well as how essential nutrients like carbon, nitrogen, and phosphorus cycle through ecosystems.
Studying Environmental Influence: Ecologists investigate how abiotic factors like temperature, water availability, soil composition, and light intensity affect the survival, reproduction, and behavior of organisms.
Predicting Ecosystem Dynamics: Ecology aims to predict how ecosystems respond to natural changes and human activities, including habitat destruction, pollution, invasive species, and global climate change.
Promoting Sustainability: Ecology provides insights for creating sustainable solutions to environmental challenges by balancing human needs with the health of natural systems.
Levels of Ecological Organization
Ecology encompasses a hierarchy of levels, each with its own focus and scope:
Organismal Ecology: Examines how individual organisms adapt and survive in their environments through physiological, behavioral, and morphological strategies.
Population Ecology: Focuses on groups of individuals of the same species, studying factors that influence population size, growth, density, and distribution.
Community Ecology: Investigates the interactions among different species living in the same area and how these interactions shape the structure and dynamics of communities.
Ecosystem Ecology: Explores the flow of energy and cycling of nutrients between organisms and their abiotic environment, emphasizing processes like primary production, decomposition, and nutrient cycling.
Landscape Ecology: Studies how ecosystems are distributed across geographic areas and how their spatial arrangements influence ecological processes.
Global Ecology (Biosphere): Examines large-scale processes that affect the Earth’s systems, such as global carbon cycling, climate regulation, and biodiversity patterns.
Historical Context and Development of Ecology
Ecology has its roots in natural history and philosophy, with early thinkers like Aristotle and Theophrastus noting the relationships between organisms and their environments. However, ecology as a distinct scientific discipline began to emerge in the 19th century.
Key milestones in its development include:
- 19th Century: The term “ecology” was coined by Ernst Haeckel in 1866, derived from the Greek words “oikos” (household) and “logos” (study), emphasizing the study of organisms’ “homes.”
- 1920s–1940s: Advances in ecosystem studies, particularly through the work of ecologists like Arthur Tansley, who introduced the concept of the “ecosystem.”
- 1960s–1970s: The rise of environmental movements highlighted the importance of ecology in addressing global issues such as deforestation, pollution, and overpopulation.
- 21st Century: Modern ecology increasingly incorporates technological advances, such as remote sensing, geographic information systems (GIS), and computer modeling, to analyze complex ecological phenomena.
Major Themes in Ecology
Biodiversity and Conservation: Understanding the diversity of life forms and the ecological roles they play is essential for conserving ecosystems and preventing species extinctions.
Climate Change and Its Impacts: Ecology examines how shifts in climate patterns affect ecosystems, species distributions, and ecosystem services like carbon storage and water filtration.
Ecosystem Services: These are the benefits that ecosystems provide to humans, including clean air, water, pollination, and soil fertility. Ecologists study how these services are maintained and how human activities threaten them.
Human Impacts on Ecosystems: Urbanization, agriculture, deforestation, pollution, and overfishing are among the human activities that disrupt ecological balance. Ecology informs strategies to mitigate these impacts.
Restoration Ecology: This emerging field focuses on repairing damaged ecosystems and restoring their functions, often through reforestation, wetland rehabilitation, and species reintroduction.
Broader Applications of Ecology
- Natural Resource Management: Ecology guides the sustainable use of forests, fisheries, and freshwater resources to ensure their availability for future generations.
- Urban Ecology: Studies how urban environments affect natural systems and explores ways to make cities more environmentally friendly.
- Agricultural Ecology: Investigates sustainable farming practices that maintain soil health, support biodiversity, and reduce environmental degradation.
- Environmental Policy and Planning: Ecological research informs policies to address environmental challenges, such as the establishment of protected areas and emission reduction targets.
- Ecological Modeling: Predictive models help forecast the impacts of environmental changes, such as species migrations in response to climate change or the spread of invasive species.
Why Study Ecology
Understanding Ecosystem Dynamics
Ecology focuses on how organisms interact with each other and their environment. It explains processes such as energy flow, nutrient cycling, and population dynamics. This understanding helps predict ecological changes and manage natural resources. It is fundamental for addressing environmental challenges.
Biodiversity and Conservation
Studying ecology helps students appreciate the importance of biodiversity for ecosystem resilience. It also teaches strategies for conserving species and habitats. This knowledge is essential for careers in wildlife management and environmental protection. It fosters a sense of responsibility toward sustaining life on Earth.
Human Impact on the Environment
Ecology helps students understand how human activities influence ecosystems through pollution, deforestation, and climate change. This knowledge informs policies and practices that promote sustainability. It also encourages students to engage in environmental advocacy. Understanding our ecological footprint is vital for creating a sustainable future.
Applications in Agriculture and Urban Planning
Ecological principles are applied to improve crop yields, manage pests, and design green urban spaces. Understanding the balance between human development and ecological health supports sustainable innovation. Students learn to think holistically and apply scientific reasoning. This prepares them for interdisciplinary careers in environmental science and planning.
Fieldwork and Research Skills
Ecology often involves outdoor fieldwork and environmental data collection. Students gain experience in observation, sampling, and statistical analysis. These skills are applicable in academic research and environmental consulting. Hands-on experiences make the study of ecology both practical and rewarding.
The Future of Ecology
As humanity faces unprecedented environmental challenges, the importance of ecology has never been greater. With increasing integration of new technologies, interdisciplinary approaches, and global collaboration, the field is poised to provide innovative solutions to safeguard the planet’s ecosystems and ensure the well-being of all living organisms.
In essence, ecology offers not only a window into the natural world but also a blueprint for living harmoniously with it, ensuring the sustainability of life on Earth for generations to come.
Ecology: Review Questions with Answers
Question 1:
What are the main components of an ecosystem, and how do they interact to maintain ecological balance?
Answer:
An ecosystem comprises both biotic (living) and abiotic (non-living) components that interact to sustain ecological balance. The main components include:
Biotic Components:
- Producers (Autotrophs): Typically plants and algae that perform photosynthesis, converting solar energy into chemical energy.
- Consumers (Heterotrophs): Organisms that consume producers or other consumers for energy, including herbivores, carnivores, omnivores, and decomposers.
- Decomposers: Fungi and bacteria that break down dead organisms and waste products, recycling nutrients back into the ecosystem.
Abiotic Components:
- Sunlight: The primary energy source driving photosynthesis and influencing climate and weather patterns.
- Water: Essential for all living organisms, influencing habitat availability and biochemical processes.
- Nutrients: Elements like nitrogen, phosphorus, and potassium necessary for organism growth and metabolism.
- Temperature: Affects metabolic rates, distribution of species, and ecosystem processes.
- Soil and Minerals: Provide physical support for plants and a medium for nutrient uptake.
Interactions Maintaining Ecological Balance:
Energy Flow: Energy enters the ecosystem through photosynthesis, moves through trophic levels via food chains and webs, and is eventually lost as heat through respiration.
Nutrient Cycling: Decomposers break down organic matter, releasing nutrients back into the soil and water, making them available for producers to reuse.
Population Regulation: Predation, competition, and disease control population sizes of species, preventing overconsumption of resources.
Symbiotic Relationships: Mutualism, commensalism, and parasitism among species enhance survival and adaptation, contributing to ecosystem stability.
Feedback Mechanisms: Negative feedback loops, such as predator-prey dynamics, help maintain species populations within sustainable limits.
In summary, the intricate interactions between biotic and abiotic components ensure the flow of energy and recycling of nutrients, which are fundamental to maintaining ecological balance and the overall health of an ecosystem.
Question 2:
Explain the concept of trophic levels in an ecosystem and describe the energy transfer efficiency between these levels.
Answer:
Trophic Levels:
Trophic levels represent the hierarchical positions of organisms within a food chain based on their feeding relationships and sources of energy:
Primary Producers (First Trophic Level): Autotrophs, mainly plants and algae, that convert solar energy into chemical energy through photosynthesis.
Primary Consumers (Second Trophic Level): Herbivores that feed directly on primary producers.
Secondary Consumers (Third Trophic Level): Carnivores or omnivores that consume primary consumers.
Tertiary Consumers (Fourth Trophic Level): Apex predators that feed on secondary consumers.
Decomposers: Although not always depicted in trophic level diagrams, decomposers break down dead organisms and waste, recycling nutrients back into the ecosystem.
Energy Transfer Efficiency:
- 10% Rule: Typically, only about 10% of the energy from one trophic level is transferred to the next. This low efficiency is due to several factors:
- Respiration: Organisms use energy for metabolic processes, releasing it as heat.
- Incomplete Consumption: Not all parts of the prey are consumed or digestible.
- Heat Loss: Energy is lost to the environment as heat during metabolic activities.
- Movement and Growth: Energy is expended in locomotion and building body tissues.
Implications of Energy Transfer Efficiency:
Energy Pyramid: Illustrates the decreasing energy available at higher trophic levels, explaining why there are usually fewer predators than prey in an ecosystem.
Biomass Distribution: Primary producers have the greatest biomass, which decreases with each subsequent trophic level due to energy loss.
Ecological Limits: The 10% efficiency limits the number of trophic levels in an ecosystem, as energy availability diminishes with each level, making it unsustainable to support numerous higher-level consumers.
Conclusion:
Understanding trophic levels and energy transfer efficiency is crucial for analyzing ecosystem structure, predicting the impact of species removal or introduction, and managing natural resources effectively. The 10% rule underscores the importance of primary producers in sustaining the entire food web.
Question 3:
Describe the carbon cycle and its significance in regulating Earth’s climate.
Answer:
Carbon Cycle Overview:
The carbon cycle is the series of processes through which carbon atoms circulate between the atmosphere, hydrosphere, biosphere, and geosphere. It involves both biological and geological mechanisms that regulate carbon distribution and maintain its balance in the environment.
Major Components of the Carbon Cycle:
Photosynthesis:
- Process: Plants, algae, and some bacteria absorb carbon dioxide (CO₂) from the atmosphere and convert it into organic compounds (glucose) using sunlight.
- Significance: Removes CO₂ from the atmosphere, reducing greenhouse gas concentrations and contributing to plant growth.
Respiration:
- Process: Organisms consume organic matter for energy, releasing CO₂ back into the atmosphere through cellular respiration.
- Significance: Balances the carbon fixed during photosynthesis by returning CO₂ to the atmosphere.
Decomposition:
- Process: Decomposers break down dead organic matter, releasing CO₂ and methane (CH₄) into the atmosphere.
- Significance: Returns carbon to the atmosphere and recycles nutrients for ecosystem use.
Oceanic Absorption and Release:
- Absorption: Oceans absorb CO₂ from the atmosphere, forming carbonic acid and bicarbonate ions.
- Release: CO₂ is released back into the atmosphere through gas exchange, influenced by temperature and biological activity.
- Significance: Acts as a major carbon sink, mitigating atmospheric CO₂ levels.
Carbonate Sedimentation:
- Process: Marine organisms use bicarbonate ions to form calcium carbonate (CaCO₃) shells and skeletons, which settle to the ocean floor upon death.
- Significance: Long-term sequestration of carbon in sedimentary rocks like limestone.
Volcanic Activity and Weathering:
- Volcanic Emissions: Release CO₂ from the Earth’s interior into the atmosphere.
- Weathering of Rocks: Chemical reactions between CO₂ and minerals on Earth’s surface form bicarbonates, which are transported to oceans.
- Significance: Provides a geological feedback mechanism regulating atmospheric CO₂ over geological timescales.
Fossil Fuel Combustion:
- Process: Burning of coal, oil, and natural gas releases stored carbon back into the atmosphere as CO₂.
- Significance: Accelerates the carbon cycle, increasing atmospheric CO₂ levels and contributing to climate change.
Significance in Regulating Earth’s Climate:
Greenhouse Effect: CO₂ is a potent greenhouse gas that traps heat in the atmosphere, maintaining Earth’s temperature. However, excessive CO₂ from human activities intensifies the greenhouse effect, leading to global warming.
Climate Regulation: The carbon cycle naturally regulates atmospheric CO₂ levels, balancing sources and sinks to stabilize climate. Disruptions to this balance can lead to climate instability.
Feedback Mechanisms:
- Positive Feedback: Warming can increase respiration rates and reduce oceanic CO₂ absorption, further elevating atmospheric CO₂.
- Negative Feedback: Enhanced plant growth from higher CO₂ can increase carbon sequestration, mitigating some warming effects.
Conclusion:
The carbon cycle is integral to maintaining Earth’s climate balance by regulating atmospheric CO₂ levels. Human-induced alterations, such as fossil fuel burning and deforestation, disrupt this cycle, enhancing the greenhouse effect and driving climate change. Understanding and managing the carbon cycle is essential for developing strategies to mitigate global warming and sustain environmental health.
Question 4:
What is biodiversity, and why is it important for ecosystem resilience and function?
Answer:
Biodiversity Defined:
Biodiversity refers to the variety and variability of life on Earth, encompassing the diversity of species, genetic variation within species, and the variety of ecosystems. It is often categorized into three levels:
Genetic Diversity: The variation of genes within a species, allowing populations to adapt to changing environments and resist diseases.
Species Diversity: The number and relative abundance of different species within a given area or ecosystem.
Ecosystem Diversity: The variety of ecosystems in a region, including forests, wetlands, deserts, and coral reefs, each supporting distinct communities of organisms.
Importance of Biodiversity for Ecosystem Resilience and Function:
Ecosystem Stability and Resilience:
- Redundancy: Multiple species often perform similar ecological roles, ensuring that ecosystem functions continue even if some species decline or go extinct.
- Response Diversity: Different species respond uniquely to environmental changes, allowing ecosystems to adapt and recover from disturbances like climate shifts, natural disasters, or human activities.
Enhanced Productivity:
- Niche Complementarity: Diverse species utilize resources more efficiently and occupy different ecological niches, leading to higher overall productivity.
- Pollination and Seed Dispersal: A variety of pollinators and seed dispersers facilitate plant reproduction and genetic diversity, sustaining plant communities and the animals that depend on them.
Nutrient Cycling and Soil Health:
- Decomposition: Diverse decomposers break down organic matter more effectively, recycling nutrients and maintaining soil fertility.
- Soil Structure: Varied root systems from different plant species enhance soil structure, promoting water retention and preventing erosion.
Provision of Ecosystem Services:
- Provisioning Services: Biodiversity ensures the availability of resources like food, medicine, and raw materials.
- Regulating Services: Diverse ecosystems regulate climate, control pests, and purify air and water.
- Cultural Services: Biodiversity contributes to cultural values, recreation, and aesthetic enjoyment.
Genetic Resources and Adaptation:
- Crop Diversity: Genetic variation in wild relatives of crops provides essential traits for breeding programs, enhancing crop resilience to pests, diseases, and environmental stresses.
- Medicinal Resources: Many pharmaceuticals are derived from diverse plant and animal species, offering potential treatments for various diseases.
Buffer Against Environmental Changes:
- Climate Regulation: Diverse forests and oceans play significant roles in carbon sequestration, mitigating climate change impacts.
- Disaster Mitigation: Wetlands and mangroves, rich in biodiversity, act as natural buffers against floods, storms, and tsunamis, protecting human communities and maintaining ecological balance.
Threats to Biodiversity:
Habitat Destruction: Deforestation, urbanization, and land conversion reduce habitat availability for many species.
Climate Change: Alters habitats and disrupts ecological relationships, leading to shifts in species distributions and interactions.
Pollution: Contaminants degrade habitats and directly harm organisms, reducing species survival rates.
Overexploitation: Excessive hunting, fishing, and harvesting deplete species populations beyond sustainable levels.
Invasive Species: Non-native species can outcompete, prey on, or bring diseases to native species, disrupting ecosystem dynamics.
Conclusion:
Biodiversity is fundamental to the health, resilience, and functionality of ecosystems. It underpins ecosystem services essential for human survival and well-being, enhances adaptability to environmental changes, and maintains the intricate balance of life on Earth. Protecting and preserving biodiversity is critical for sustaining ecosystems and ensuring a stable and prosperous future for all living organisms.
Question 5:
Define ecological succession and differentiate between primary and secondary succession, including their driving factors and typical stages.
Answer:
Ecological Succession Defined:
Ecological succession is the gradual, directional change in the species composition and structure of an ecological community over time. It leads to the establishment of a stable, climax community that is well-adapted to the prevailing environmental conditions.
Types of Succession:
Primary Succession:
Definition: Occurs in lifeless areas where no soil initially exists, such as bare rock surfaces, lava flows, or regions exposed by glacial retreat.
Driving Factors:
- Initial Colonization: Pioneer species, typically hardy organisms like lichens and certain mosses, colonize the barren substrate.
- Soil Formation: These pioneers contribute to soil development by breaking down rock through physical and chemical means, and by accumulating organic matter as they grow and die.
- Environmental Gradients: Gradual changes in moisture, temperature, and nutrient availability facilitate the establishment of successive plant and animal communities.
Typical Stages:
- Pioneer Stage: Dominated by pioneer species that can tolerate harsh conditions and initiate soil formation.
- Intermediate Stages: Grasses, herbs, and shrubs begin to establish as soil depth and quality improve.
- Climax Community: A stable, mature community (e.g., forest) that remains relatively unchanged unless disturbed by external factors.
Secondary Succession:
Definition: Occurs in areas where a community previously existed but was removed by a disturbance, leaving the soil intact. Examples include areas after forest fires, agricultural abandonment, or hurricanes.
Driving Factors:
- Disturbance: An event disrupts the existing community but does not eliminate the soil, allowing for quicker recolonization.
- Seed Bank and Residual Organisms: Seeds present in the soil and surviving organisms facilitate rapid re-establishment of plant and animal communities.
- Resource Availability: Existing soil nutrients and structure support faster growth and recovery of species.
Typical Stages:
- Pioneer Stage: Fast-growing, weedy species (e.g., grasses, herbs) quickly colonize the area, stabilizing the soil.
- Intermediate Stages: Shrubs and young trees replace pioneer species as conditions become more favorable.
- Climax Community: A mature forest or other stable community re-establishes, similar to the original pre-disturbance community.
Differences Between Primary and Secondary Succession:
Initial Conditions:
- Primary Succession: Starts on barren substrates without soil.
- Secondary Succession: Begins in areas with existing soil and remnants of the previous community.
Pioneer Species:
- Primary Succession: Typically lichens, mosses, and hardy grasses.
- Secondary Succession: Usually fast-growing herbaceous plants and shrubs.
Pace of Succession:
- Primary Succession: Generally slower due to initial soil formation.
- Secondary Succession: Faster as soil and seed banks are already present.
Stages and Complexity:
- Primary Succession: Involves more gradual development of soil and complex communities.
- Secondary Succession: Progresses more rapidly to complex communities, often reaching climax stages quicker.
Conclusion:
Ecological succession is a fundamental process that shapes the structure and composition of ecological communities over time. Understanding the differences between primary and secondary succession helps in managing ecosystems, restoring disturbed areas, and predicting how communities will respond to various environmental changes and disturbances.
Question 6:
What are keystone species, and how do they influence the structure and diversity of ecological communities? Provide examples.
Answer:
Keystone Species Defined:
A keystone species is a species that has a disproportionately large impact on its ecological community relative to its abundance. These species play critical roles in maintaining the structure, diversity, and function of ecosystems, often by influencing the population sizes and interactions of other species.
Influence on Ecological Communities:
Regulating Predator-Prey Relationships:
- Top Predators: Control the populations of herbivores or other predators, preventing overgrazing or cascading trophic effects that can alter habitat structure and species composition.
Facilitating Species Diversity:
- Mutualists: Enhance the survival and reproduction of other species through symbiotic relationships, promoting higher biodiversity.
- Ecosystem Engineers: Modify the physical environment in ways that create niches for other organisms (e.g., beavers building dams).
Maintaining Habitat Structure:
- Primary Producers: Some plants or algae form the foundational structure of habitats, supporting various consumer species.
Preventing Competitive Exclusion:
- Dominant Competitors: Keep certain species in check, allowing a variety of species to coexist without any single species monopolizing resources.
Examples of Keystone Species:
Sea Otters (Enhydra lutris):
- Role: Predate on sea urchins, preventing overgrazing of kelp forests.
- Impact: Kelp forests provide habitat and food for numerous marine species. Without sea otters, sea urchin populations explode, decimating kelp forests and reducing marine biodiversity.
African Elephants (Loxodonta africana):
- Role: Modify vegetation by uprooting trees and trampling shrubs.
- Impact: Create grasslands from forests, supporting species adapted to open habitats. Their activities prevent forest encroachment, maintaining savanna ecosystems rich in biodiversity.
Beavers (Castor canadensis):
- Role: Construct dams, creating ponds and wetlands.
- Impact: Wetlands serve as habitats for diverse aquatic and terrestrial species. Beaver activity increases water availability, enhances nutrient cycling, and provides breeding grounds for various organisms.
Sea Stars (Pisaster ochraceus):
- Role: Prey on mussels, which are dominant competitors in intertidal zones.
- Impact: By controlling mussel populations, sea stars allow a variety of other invertebrates and algae to thrive, increasing intertidal biodiversity.
Wolves (Canis lupus):
- Role: Apex predators that regulate ungulate populations.
- Impact: In areas like Yellowstone National Park, wolf reintroduction has led to reduced elk populations, which in turn allowed vegetation recovery, benefiting multiple species and restoring ecosystem balance.
Honeybees (Apis mellifera):
- Role: Pollinators for a wide range of flowering plants.
- Impact: Facilitate plant reproduction, ensuring the survival of plant species and the animals that depend on them, thereby maintaining ecosystem productivity and diversity.
Consequences of Keystone Species Loss:
Trophic Cascades: The removal of a keystone species can trigger a cascade of changes across trophic levels, leading to significant alterations in community structure and reduced biodiversity.
Habitat Degradation: Loss of ecosystem engineers can lead to habitat loss or degradation, negatively impacting numerous dependent species.
Increased Vulnerability: Ecosystems become more susceptible to invasions by non-native species and less resilient to environmental changes and disturbances.
Conclusion:
Keystone species are essential for maintaining the balance and diversity of ecological communities. Their unique roles ensure the stability and functionality of ecosystems, making their conservation critical for preserving biodiversity and ecosystem health. Understanding the importance of keystone species aids in effective ecosystem management and restoration efforts.
Question 7:
Explain the concept of ecological niches and the differences between fundamental and realized niches with examples.
Answer:
Ecological Niche Defined:
An ecological niche encompasses the role and position a species has in its environment, including how it obtains resources, interacts with other organisms, and responds to environmental conditions. It describes the functional role of a species within an ecosystem.
Fundamental vs. Realized Niches:
- Fundamental Niche:
- Definition: The full range of environmental conditions and resources an organism can potentially utilize without considering biotic interactions such as competition, predation, or disease.
- Characteristics:
- Represents the potential niche based solely on abiotic factors like temperature, humidity, soil type, and available resources.
- Broader and encompasses all the conditions under which a species can survive and reproduce.
- Realized Niche:
- Definition: The actual set of environmental conditions and resources a species utilizes in nature, considering biotic interactions and competition.
- Characteristics:
- Typically narrower than the fundamental niche due to limitations imposed by interactions with other species.
- Reflects the optimal conditions where the species can thrive amidst competition and predation.
Examples Illustrating the Difference:
Tree Species in a Forest:
- Fundamental Niche: A tree species might have the potential to grow in a wide range of light, soil, and moisture conditions.
- Realized Niche: In the forest, light availability is limited by canopy cover, and competition for nutrients with other trees reduces the range of conditions where the species can successfully establish and grow.
Barn Owl (Tyto alba):
- Fundamental Niche: Can potentially inhabit a variety of habitats where prey (small mammals) is available, including grasslands, forests, and urban areas.
- Realized Niche: Prefers open areas like grasslands and agricultural fields where hunting is more efficient and competition with other predators is lower.
Darwin’s Finches:
- Fundamental Niche: Each finch species could exploit a range of seed sizes and types.
- Realized Niche: Due to competition among finch species, each species specializes in specific seed types, reducing direct competition and allowing coexistence.
Marine Phytoplankton:
- Fundamental Niche: Capable of surviving across a range of light and nutrient conditions in the ocean.
- Realized Niche: Limited to specific depths and nutrient-rich areas where competition with other phytoplankton species is minimized.
Factors Influencing the Difference Between Niches:
Competition: Presence of other species competing for the same resources can constrain a species’ realized niche.
Predation and Parasitism: Threats from predators and parasites can limit where a species can safely exist.
Mutualism and Symbiosis: Positive interactions with other species can expand a species’ realized niche by providing additional resources or protection.
Human Activities: Habitat alteration, pollution, and other anthropogenic factors can alter both fundamental and realized niches.
Conclusion:
Understanding the distinction between fundamental and realized niches is crucial for studying species distribution, community structure, and ecosystem dynamics. It highlights the impact of biotic interactions on the actual living conditions of species and aids in predicting how changes in the environment or species interactions might affect biodiversity and ecosystem functionality.
Question 8:
Discuss the role of keystone predators in regulating ecosystem dynamics and provide examples of their impact on biodiversity.
Answer:
Keystone Predators Defined:
Keystone predators are apex predators that have a significant, disproportionate influence on their ecosystem relative to their abundance. They regulate the populations of other species, maintain species diversity, and structure the community by controlling trophic cascades.
Role in Regulating Ecosystem Dynamics:
Population Control:
- Predator-Prey Balance: Keystone predators keep herbivore populations in check, preventing overgrazing and allowing vegetation and other species to thrive.
- Prey Selection: They may preferentially consume certain prey species, influencing the community structure and promoting biodiversity by preventing any single prey species from dominating.
Trophic Cascades:
- Top-Down Regulation: The presence of a keystone predator can initiate a trophic cascade, where effects trickle down through lower trophic levels, influencing the entire ecosystem.
- Indirect Interactions: By controlling herbivore numbers, keystone predators indirectly affect plant community composition and the species that depend on those plants.
Habitat Modification:
- Behavioral Changes: Keystone predators can alter the behavior of prey species, such as reducing their feeding rates or changing their habitat use, which in turn affects vegetation and other animal populations.
- Ecosystem Engineering: Some keystone predators modify habitats (e.g., beavers), creating environments that support diverse species.
Maintaining Biodiversity:
- Preventing Competitive Exclusion: By regulating dominant species, keystone predators ensure that multiple species can coexist, enhancing overall biodiversity.
- Supporting Mutualistic Relationships: Healthy predator populations can sustain mutualistic interactions among other species, such as pollination and seed dispersal.
Examples of Keystone Predators and Their Impact on Biodiversity:
Sea Otters (Enhydra lutris) in Kelp Forests:
- Role: Predate on sea urchins, controlling their populations.
- Impact: Prevent overgrazing by sea urchins on kelp forests. Healthy kelp forests provide habitat and food for numerous marine species, enhancing biodiversity.
- Consequences of Removal: Without sea otters, sea urchin populations explode, decimating kelp forests and leading to a decline in marine biodiversity.
Wolves (Canis lupus) in Yellowstone National Park:
- Role: Apex predators that regulate elk populations.
- Impact: Reduced elk numbers allow vegetation, such as willow and aspen, to recover. This leads to increased biodiversity, including songbirds, beavers, and other species dependent on riparian habitats.
- Consequences of Removal: Elk overpopulation leads to overgrazing, trampling of vegetation, and reduced habitat for other species, resulting in decreased biodiversity.
Sea Stars (Pisaster ochraceus) in Intertidal Zones:
- Role: Predate on mussels, which are competitive dominant species.
- Impact: Control mussel populations, allowing for greater species diversity by enabling other invertebrates and algae to coexist.
- Consequences of Removal: Mussel populations dominate, outcompeting and reducing the diversity of other intertidal species.
African Wild Dogs (Lycaon pictus) in Savannas:
- Role: Predate on various herbivores, preventing any single prey species from becoming too abundant.
- Impact: Maintains balance among herbivore populations, promoting a diverse and stable ecosystem.
- Consequences of Decline: Overabundance of certain herbivores can lead to overgrazing, habitat degradation, and reduced biodiversity.
Lions (Panthera leo) in African Grasslands:
- Role: Regulate populations of large herbivores like wildebeest and zebras.
- Impact: Prevent overgrazing, allowing grasses and other vegetation to flourish, which supports a variety of other species.
- Consequences of Decline: Overgrazing by herbivores can lead to habitat loss for numerous species, decreasing ecosystem diversity.
Consequences of Keystone Predator Loss:
Ecosystem Imbalance: Removal of keystone predators disrupts the balance of species populations, leading to overpopulation of certain species and decline of others.
Reduced Biodiversity: Dominance of certain species due to lack of predation can reduce species richness and overall biodiversity.
Habitat Degradation: Overgrazing or overpopulation of herbivores can lead to habitat loss, erosion, and reduced ecosystem services.
Conclusion:
Keystone predators play a crucial role in maintaining the structure, diversity, and functionality of ecological communities. Their influence extends beyond direct predation, affecting trophic interactions, habitat structure, and species coexistence. Conservation and protection of keystone predators are essential for preserving biodiversity and ensuring the resilience and health of ecosystems.
Question 9:
What are invasive species, and how do they affect native biodiversity and ecosystem functioning? Provide specific examples.
Answer:
Invasive Species Defined:
Invasive species are non-native organisms introduced to a new environment, where they establish, spread rapidly, and cause harm to native species, ecosystems, human health, or economies. Unlike native species, invasive species often lack natural predators or controls in their new habitats, allowing them to proliferate unchecked.
Effects on Native Biodiversity and Ecosystem Functioning:
Competition:
- Resource Competition: Invasive species often outcompete native species for essential resources such as food, light, water, and space.
- Reproductive Dominance: They may reproduce more quickly or efficiently than native species, reducing native populations.
Predation and Herbivory:
- Direct Predation: Invasive predators can decimate native prey populations, leading to declines or extinctions.
- Herbivory Pressure: Invasive herbivores may overgraze native plants, disrupting plant communities and associated species.
Habitat Alteration:
- Physical Changes: Some invasive species modify habitats, making them unsuitable for native species. For example, invasive plants can alter soil chemistry or hydrology.
- Ecosystem Engineering: Species like beavers or certain invasive plants can change the physical structure of ecosystems, impacting native biodiversity.
Disease Introduction:
- Pathogen Transmission: Invasive species can introduce new diseases or parasites to which native species have no resistance, causing population declines.
Hybridization:
- Genetic Mixing: Invasive species can interbreed with closely related native species, leading to hybrid offspring that may outcompete pure native strains.
Disruption of Mutualistic Relationships:
- Pollination and Seed Dispersal: Invasive species may interfere with native pollinators or seed dispersers, affecting plant reproduction and ecosystem dynamics.
Examples of Invasive Species and Their Impacts:
Zebra Mussels (Dreissena polymorpha) in North American Waterways:
- Impact: Outcompete native mussels for food and space, clog water intake pipes, disrupt local aquatic food webs, and alter nutrient cycling.
- Consequences: Reduction in native mussel populations, economic costs for water infrastructure maintenance, and changes in aquatic ecosystem structure.
European Starling (Sturnus vulgaris) in North America:
- Impact: Compete with native bird species for nesting sites and food resources, leading to declines in native bird populations.
- Consequences: Decreased biodiversity among native birds and potential disruptions in ecosystem functions like insect control.
Cane Toads (Rhinella marina) in Australia:
- Impact: Poisonous to native predators, reducing populations of species that attempt to consume them. They also compete with native amphibians for resources.
- Consequences: Declines in native predator species, disruption of amphibian communities, and alteration of ecosystem dynamics.
Asian Carp (Hypophthalmichthys molitrix) in North American Rivers:
- Impact: Outcompete native fish for planktonic food resources, alter aquatic vegetation, and disrupt food webs.
- Consequences: Declines in native fish populations, economic impacts on fisheries, and changes in river ecosystem structure.
Kudzu (Pueraria montana) in the Southeastern United States:
- Impact: Rapidly grows over native vegetation, blocking sunlight and smothering plants. It also alters soil nitrogen levels.
- Consequences: Loss of native plant diversity, changes in habitat structure, and impacts on wildlife dependent on native flora.
Lionfish (Pterois volitans and Pterois miles) in the Western Atlantic and Caribbean:
- Impact: Predate on native fish and invertebrates, reducing populations of species important for coral reef health.
- Consequences: Declines in reef fish diversity, altered reef community structure, and potential impacts on reef resilience.
Management and Control of Invasive Species:
Prevention: Implementing biosecurity measures to prevent the introduction and spread of invasive species, such as inspections, quarantine, and public education.
Early Detection and Rapid Response: Monitoring ecosystems for new invasions and acting swiftly to eradicate or control invasive populations before they become established.
Biological Control: Introducing natural predators, parasites, or pathogens to control invasive species, though this method requires careful assessment to avoid unintended consequences.
Mechanical and Chemical Control: Physically removing invasive species or using herbicides and pesticides to reduce their populations, which may have collateral effects on native species.
Restoration Ecology: Rehabilitating ecosystems by reintroducing native species and restoring natural processes after invasive species have been controlled.
Conclusion:
Invasive species pose significant threats to native biodiversity and ecosystem functioning by altering species interactions, disrupting food webs, and modifying habitats. Effective management requires a combination of prevention, early detection, control measures, and ecosystem restoration to mitigate their impacts and preserve ecological integrity.
Question 10:
Describe the concept of ecosystem services and categorize them into provisioning, regulating, supporting, and cultural services with examples.
Answer:
Ecosystem Services Defined:
Ecosystem services are the benefits that humans derive from ecosystems, essential for survival, well-being, and economic activities. These services arise from the complex interactions within ecosystems, supporting life and providing resources and environmental regulation.
Categories of Ecosystem Services:
Provisioning Services:
- Definition: Products obtained from ecosystems that directly satisfy human needs.
- Examples:
- Food: Harvesting of crops, livestock, fish, and wild game.
- Water: Supply of fresh water for drinking, irrigation, and sanitation.
- Raw Materials: Timber, fiber, and biofuels used in construction, clothing, and energy production.
- Medicinal Resources: Plants and organisms that provide pharmaceuticals and traditional medicines.
- Genetic Resources: Genetic diversity for crop improvement, breeding programs, and biotechnology.
Regulating Services:
- Definition: Benefits obtained from the regulation of ecosystem processes.
- Examples:
- Climate Regulation: Carbon sequestration by forests and oceans helps mitigate climate change.
- Water Purification: Wetlands and riparian zones filter pollutants, improving water quality.
- Pollination: Insects like bees and butterflies facilitate the reproduction of many crops and wild plants.
- Pest Control: Predators and parasitoids regulate populations of agricultural pests, reducing the need for chemical pesticides.
- Flood Regulation: Mangroves, swamps, and forests absorb excess rainfall, reducing the severity of floods.
Supporting Services:
- Definition: Fundamental services necessary for the production of all other ecosystem services.
- Examples:
- Nutrient Cycling: Decomposition and mineralization processes recycle nutrients, making them available for plant growth.
- Soil Formation: Weathering of rocks and organic matter accumulation create fertile soil essential for agriculture.
- Primary Production: Photosynthesis by plants and algae forms the base of food webs, providing energy for all trophic levels.
- Habitat Provision: Ecosystems provide habitats for a diverse array of species, maintaining biodiversity.
Cultural Services:
- Definition: Non-material benefits people obtain from ecosystems through spiritual enrichment, cognitive development, reflection, recreation, and aesthetic experiences.
- Examples:
- Recreational Activities: Hiking, bird-watching, fishing, and tourism activities that provide leisure and economic benefits.
- Spiritual and Religious Values: Sacred natural sites and landscapes hold spiritual significance for various cultures and religions.
- Educational and Scientific Research: Natural environments offer opportunities for learning, research, and innovation.
- Aesthetic Appreciation: Scenic landscapes and biodiversity contribute to human happiness, creativity, and cultural identity.
- Cultural Heritage: Ecosystems play a role in traditional practices, folklore, and cultural narratives, preserving community identities.
Importance of Ecosystem Services:
Human Well-Being: Ecosystem services are fundamental to human health, survival, and quality of life, providing essential resources and regulating environmental conditions.
Economic Value: Many ecosystem services underpin economic activities, agriculture, fisheries, tourism, and other industries, contributing significantly to global economies.
Sustainability: Maintaining ecosystem services ensures the sustainability of natural resources and the resilience of ecosystems against environmental changes and disturbances.
Biodiversity Conservation: Protecting ecosystem services often involves conserving biodiversity, as diverse ecosystems are more capable of providing a wide range of services.
Challenges in Ecosystem Services:
Valuation: Quantifying and monetizing ecosystem services can be complex, hindering their integration into decision-making processes.
Trade-offs: Enhancing certain ecosystem services may lead to the decline of others, requiring balanced management approaches.
Degradation and Loss: Human activities like deforestation, pollution, and climate change threaten ecosystem services, leading to diminished benefits for society.
Policy Integration: Incorporating ecosystem services into policies and governance frameworks is essential for their protection and sustainable use.
Conclusion:
Ecosystem services are integral to the functioning of both natural and human systems, providing essential benefits that sustain life and support economic activities. Recognizing and valuing these services is crucial for informed decision-making, conservation efforts, and fostering sustainable development. Protecting ecosystem services ensures the continued provision of vital resources and the maintenance of environmental health and resilience.
Question 11:
How do mutualistic relationships enhance ecosystem stability and what are some key examples of mutualism in nature?
Answer:
Mutualistic Relationships Defined:
Mutualism is a type of symbiotic relationship where both participating species derive benefits, enhancing their survival, reproduction, or overall fitness. These interactions contribute to ecosystem stability by fostering cooperation, enhancing resource utilization, and promoting biodiversity.
Enhancement of Ecosystem Stability through Mutualism:
Resource Efficiency:
- Complementary Resource Use: Mutualistic partners utilize different resources or exploit resources more efficiently together than they could individually, leading to increased productivity and resource conservation.
Biodiversity Promotion:
- Facilitating Species Coexistence: Mutualistic interactions can support niche differentiation, allowing multiple species to coexist and reducing competitive exclusion.
- Enhancing Reproductive Success: Pollination and seed dispersal mutualisms increase plant diversity, which in turn supports a variety of animal species.
Ecosystem Resilience:
- Buffering Against Disturbances: Mutualistic networks can provide redundancy, ensuring that ecosystem functions continue even if some mutualistic relationships are disrupted.
- Rapid Recovery: Mutualistic interactions can accelerate ecosystem recovery after disturbances by facilitating resource redistribution and habitat restoration.
Stabilizing Food Webs:
- Promoting Interconnectedness: Mutualism creates intricate connections among species, enhancing the complexity and stability of food webs.
- Preventing Trophic Imbalances: By supporting key species, mutualistic relationships help maintain balanced trophic interactions, preventing dominance by any single species.
Key Examples of Mutualism in Nature:
Pollination Mutualism:
- Bees and Flowers: Bees collect nectar and pollen from flowers for food, inadvertently transferring pollen from one flower to another, facilitating plant reproduction.
- Impact: Enhances plant genetic diversity, supports agricultural productivity, and maintains ecosystem plant communities.
Mycorrhizal Fungi and Plants:
- Association: Mycorrhizal fungi colonize plant roots, extending their hyphal networks into the soil.
- Benefits to Plants: Improved water and nutrient uptake, particularly phosphorus.
- Benefits to Fungi: Access to carbohydrates produced by plants through photosynthesis.
- Impact: Increases plant growth and soil health, supports diverse plant communities.
Cleaner Fish and Host Fish:
- Example: Cleaner wrasses remove parasites and dead tissue from larger host fish.
- Benefits to Host Fish: Reduced parasite load and improved health.
- Benefits to Cleaner Fish: Access to a reliable food source.
- Impact: Enhances fish health, promotes biodiversity by supporting the survival of multiple host species.
Ants and Acacia Trees:
- Mutualism: Certain ant species inhabit the hollow thorns of Acacia trees, protecting them from herbivores and competing plants.
- Benefits to Acacia Trees: Defense against herbivores and prevention of overgrowth by competing vegetation.
- Benefits to Ants: Shelter and food sources provided by the tree (e.g., nectar, protein-rich Beltian bodies).
- Impact: Maintains Acacia populations and associated biodiversity, supporting a range of other organisms dependent on Acacia habitats.
Lichens (Algae and Fungi):
- Mutualism: Algae perform photosynthesis, providing carbohydrates to the fungal partner, while fungi offer protection and access to nutrients.
- Benefits to Algae: Protection from environmental stressors and enhanced access to water and minerals.
- Benefits to Fungi: Sustained energy supply and access to atmospheric nutrients.
- Impact: Enables colonization of harsh environments, contributes to soil formation, and supports diverse ecosystems by providing foundational species.
Humans and Gut Microbiota:
- Mutualism: Gut bacteria aid in digestion, synthesize essential vitamins, and protect against pathogenic microbes.
- Benefits to Humans: Improved nutrient absorption, immune system support, and overall health.
- Benefits to Bacteria: Stable habitat and access to nutrients from the host’s diet.
- Impact: Maintains human health, influences behavior and metabolism, and contributes to disease prevention.
Coral and Zooxanthellae:
- Mutualism: Zooxanthellae (photosynthetic algae) live within coral tissues, providing energy through photosynthesis.
- Benefits to Corals: Enhanced growth and reef building through the supply of organic compounds.
- Benefits to Zooxanthellae: Protected environment and access to nutrients from coral metabolism.
- Impact: Supports the formation of coral reefs, which are hotspots of marine biodiversity and provide essential ecosystem services like coastal protection and fisheries support.
Consequences of Disrupting Mutualistic Relationships:
Ecosystem Imbalance: Loss of mutualistic partners can lead to declines in populations and disruptions of ecosystem functions.
Reduced Biodiversity: Disrupted mutualism can decrease species diversity by eliminating key interactions that support various organisms.
Vulnerability to Stressors: Ecosystems reliant on mutualistic interactions may become more susceptible to environmental changes and disturbances.
Conclusion:
Mutualistic relationships are fundamental to the structure, function, and stability of ecosystems. They enhance resource efficiency, promote biodiversity, and contribute to ecosystem resilience. Protecting and fostering mutualistic interactions is essential for maintaining healthy and sustainable ecosystems, which in turn support human well-being and biodiversity conservation.
Question 12:
How do ecological niches contribute to species coexistence, and what mechanisms prevent competitive exclusion in diverse communities?
Answer:
Ecological Niches and Species Coexistence:
Ecological niches define the role of a species within an ecosystem, encompassing how it obtains resources, interacts with other organisms, and responds to environmental conditions. Effective niche differentiation allows multiple species to occupy similar habitats without directly competing for identical resources, facilitating species coexistence.
Mechanisms Preventing Competitive Exclusion:
Niche Differentiation (Resource Partitioning):
- Definition: Species evolve to utilize different resources or the same resource in different ways, reducing direct competition.
- Examples:
- Temporal Partitioning: Different species use the same resource at different times (e.g., diurnal vs. nocturnal feeding).
- Spatial Partitioning: Species occupy different physical spaces or microhabitats within the same environment (e.g., birds nesting at varying heights in trees).
- Dietary Differentiation: Species consume different types or sizes of prey, minimizing overlap in food sources.
Competitive Exclusion Principle:
- Definition: No two species can occupy the exact same niche indefinitely in a stable environment. One species will outcompete and exclude the other.
- Prevention: Niche differentiation and other mechanisms create niche differences that allow species to coexist by reducing overlap.
Interspecific Competition:
- Role in Coexistence: Moderate levels of competition can maintain species diversity by preventing any one species from becoming overly dominant, while not driving others to extinction.
Mutualistic Interactions:
- Facilitating Coexistence: Mutualisms, such as pollination or seed dispersal relationships, can enhance species coexistence by providing mutual benefits that reduce direct competition for resources.
Predation Pressure:
- Keystone Predators: Predators that regulate prey populations can prevent competitive exclusion by maintaining balanced populations and allowing multiple prey species to coexist.
Environmental Heterogeneity:
- Variable Conditions: Diverse and changing environmental conditions create multiple niches and refuges where different species can thrive, reducing direct competition.
Neutral Theory:
- Random Events: Some theories suggest that stochastic events and random dispersal can allow species to coexist despite similar niches, especially in highly diverse communities.
Adaptive Traits and Evolutionary Changes:
- Rapid Adaptation: Species can quickly adapt to changing conditions or develop new traits that allow them to exploit different resources or habitats, promoting coexistence.
Examples Illustrating Species Coexistence:
Darwin’s Finches on the Galápagos Islands:
- Niche Differentiation: Finch species have evolved distinct beak shapes and sizes tailored to different food sources (e.g., seeds, insects), reducing competition and allowing multiple species to coexist on the same islands.
African Savannah Grazers:
- Spatial and Dietary Partitioning: Different herbivore species (e.g., zebras, wildebeest, gazelles) graze on varying types of vegetation and in different areas of the savannah, minimizing direct competition.
Coral Reef Fish:
- Microhabitat Utilization: Various fish species occupy different niches within the coral reef structure, such as different zones (e.g., crests, lagoons) and feeding strategies, promoting high biodiversity.
Forest Bird Species:
- Temporal Partitioning: Some birds forage during the day while others are nocturnal, allowing similar species to utilize the same habitat without direct competition for food resources.
Consequences of Competitive Exclusion:
Species Decline or Extinction: Without mechanisms to promote coexistence, dominant species can outcompete and drive other species to local extinction.
Reduced Biodiversity: Competitive exclusion can lead to lower species richness and diversity, weakening ecosystem resilience and functionality.
Importance of Understanding Niche Dynamics:
Conservation Efforts: Recognizing how niches support species coexistence aids in habitat management and restoration, ensuring the preservation of diverse communities.
Predicting Ecosystem Responses: Understanding niche interactions helps predict how ecosystems might respond to changes such as species introductions, climate change, or habitat alteration.
Conclusion
Ecological niches are fundamental to the coexistence of species within ecosystems by promoting resource specialization and reducing direct competition. Mechanisms like niche differentiation, mutualistic interactions, and environmental heterogeneity prevent competitive exclusion, maintaining species diversity and ecosystem stability. Understanding these dynamics is essential for conserving biodiversity and managing ecosystems effectively.
Ecology: Thought-Provoking Questions
1. How do energy pyramids illustrate the flow of energy in ecosystems, and what factors influence energy transfer efficiency between trophic levels?
Answer:
Energy Pyramids Defined:
Energy pyramids are graphical representations that depict the distribution of energy among different trophic levels within an ecosystem. They visually demonstrate how energy diminishes as it moves from producers at the base to various levels of consumers above.
Structure of Energy Pyramids:
Base Level (Primary Producers): This level consists of autotrophic organisms, primarily plants and algae, that harness energy from the sun through photosynthesis to produce organic compounds.
Intermediate Levels (Consumers): These include primary consumers (herbivores), secondary consumers (carnivores that eat herbivores), and tertiary consumers (carnivores that eat other carnivores).
Apex Level (Top Predators): The highest level typically features apex predators that have few or no natural enemies.
Flow of Energy:
Photosynthesis: Primary producers convert solar energy into chemical energy stored in glucose and other organic molecules.
Consumption: Primary consumers ingest the producers, obtaining energy by digesting plant tissues. Secondary and tertiary consumers continue this process up the trophic levels.
Respiration and Heat Loss: At each trophic level, organisms use a portion of the consumed energy for metabolic processes, growth, and reproduction. A significant amount of energy is lost as heat due to the second law of thermodynamics.
Energy Transfer Efficiency:
- 10% Rule: On average, only about 10% of the energy from one trophic level is transferred to the next. This low efficiency is attributed to:
- Metabolic Processes: Energy is expended during activities such as movement, growth, and reproduction.
- Incomplete Consumption: Not all parts of the prey are consumed or digestible.
- Heat Loss: Energy dissipates as heat through respiration.
Factors Influencing Energy Transfer Efficiency:
Organism Size and Metabolism:
- Larger organisms often have lower energy transfer efficiency due to higher energy demands.
- High metabolic rates can increase energy expenditure, reducing the energy available for transfer.
Diet Composition:
- Carnivores typically have lower energy transfer efficiency compared to herbivores because they consume less energy-dense food sources.
Ecological Adaptations:
- Some organisms have adaptations that enhance energy retention, such as fat storage in animals or efficient photosynthetic pathways in plants.
Environmental Conditions:
- Availability of resources can influence how much energy is captured and transferred. Scarce resources may lead to lower energy transfer rates.
Trophic Level Diversity:
- Ecosystems with multiple intermediate trophic levels may experience compounded energy losses, limiting the number of viable higher trophic levels.
Implications of Energy Pyramids:
Ecosystem Productivity: Energy pyramids help in understanding the overall productivity of ecosystems and the biomass distribution among trophic levels.
Biodiversity Support: Limited energy at higher trophic levels constrains the number of apex predators and influences species diversity.
Conservation Efforts: Understanding energy flow aids in identifying key species and interactions that sustain ecosystem health.
Conclusion:
Energy pyramids are fundamental tools in ecology that illustrate the diminishing energy as it flows through trophic levels. The efficiency of energy transfer is influenced by various biological and environmental factors, shaping the structure and dynamics of ecosystems. Recognizing these patterns is essential for studying ecosystem productivity, species interactions, and the impacts of environmental changes.
2. What are the main biogeochemical cycles, and why are they essential for ecosystem functioning?
Answer:
Biogeochemical Cycles Defined:
Biogeochemical cycles are natural processes that recycle essential elements and compounds between living organisms (biosphere) and non-living components (geosphere, hydrosphere, and atmosphere) of the Earth. These cycles ensure the continuous availability of vital nutrients necessary for life.
Main Biogeochemical Cycles:
Carbon Cycle:
- Components: Carbon dioxide (CO₂), organic carbon, methane (CH₄).
- Processes: Photosynthesis, respiration, decomposition, combustion, ocean absorption, sedimentation.
- Importance: Regulates Earth’s climate by controlling greenhouse gas concentrations; essential for the formation of organic molecules in living organisms.
Nitrogen Cycle:
- Components: Atmospheric nitrogen (N₂), ammonia (NH₃), nitrates (NO₃⁻), nitrites (NO₂⁻).
- Processes: Nitrogen fixation, nitrification, denitrification, ammonification, assimilation.
- Importance: Provides nitrogen, a critical component of amino acids and nucleic acids, to living organisms; influences soil fertility and plant growth.
Phosphorus Cycle:
- Components: Phosphate ions (PO₄³⁻), organic phosphorus compounds.
- Processes: Weathering of rocks, uptake by organisms, decomposition, sedimentation.
- Importance: Essential for DNA, RNA, ATP, and phospholipids; crucial for energy transfer and genetic information in cells.
Sulfur Cycle:
- Components: Sulfur dioxide (SO₂), hydrogen sulfide (H₂S), sulfate ions (SO₄²⁻).
- Processes: Volcanic emissions, oxidation, reduction, assimilation, mineralization.
- Importance: Integral to amino acids like cysteine and methionine; affects soil acidity and plant nutrient availability.
Water Cycle (Hydrological Cycle):
- Components: Water (H₂O) in various states (solid, liquid, gas).
- Processes: Evaporation, condensation, precipitation, infiltration, runoff, transpiration.
- Importance: Distributes water resources, supports all forms of life, regulates climate, shapes geological features.
Oxygen Cycle:
- Components: Molecular oxygen (O₂), ozone (O₃).
- Processes: Photosynthesis, respiration, photodissociation, combustion.
- Importance: Essential for aerobic respiration in most organisms; protects Earth from harmful ultraviolet radiation.
Why Biogeochemical Cycles Are Essential:
- Nutrient Availability:
- Continuous Supply: These cycles ensure that essential elements are continuously recycled and made available to organisms, preventing depletion of vital nutrients.
- Ecosystem Stability:
- Homeostasis: Biogeochemical cycles maintain the balance of elements, supporting stable and resilient ecosystems that can withstand environmental fluctuations.
- Climate Regulation:
- Greenhouse Gases: Cycles like the carbon and sulfur cycles regulate atmospheric concentrations of greenhouse gases, influencing global temperatures and climate patterns.
- Soil Fertility:
- Nutrient Recycling: The nitrogen and phosphorus cycles replenish soil nutrients, promoting plant growth and agricultural productivity.
- Water Quality and Distribution:
- Purification Processes: The water cycle and related biogeochemical processes help in purifying water, distributing it across various ecosystems, and maintaining hydrological balance.
- Support for Life Processes:
- Biological Functions: Elements like carbon, nitrogen, and phosphorus are fundamental to biological molecules and processes, enabling life to thrive.
- Geological Impacts:
- Rock Formation and Erosion: Biogeochemical cycles influence geological processes such as rock weathering, sedimentation, and mineral formation, shaping Earth’s surface.
Interconnectedness of Cycles:
Biogeochemical cycles are interdependent, with changes in one cycle often affecting others. For example:
- Carbon and Nitrogen Cycles: Nitrogen availability can influence plant growth, affecting carbon sequestration through photosynthesis.
- Water and Phosphorus Cycles: Water movement aids in the transport of phosphorus from soil to water bodies, influencing aquatic ecosystems.
Human Impact on Biogeochemical Cycles:
Human activities have significantly altered these natural cycles, leading to:
- Carbon Cycle: Increased CO₂ emissions from fossil fuel combustion contribute to global warming and climate change.
- Nitrogen Cycle: Excessive use of nitrogen-based fertilizers leads to eutrophication and water pollution.
- Phosphorus Cycle: Mining and runoff of phosphate fertilizers cause nutrient overload in aquatic systems.
- Sulfur Cycle: Industrial emissions of SO₂ lead to acid rain, damaging ecosystems and structures.
- Water Cycle: Urbanization and deforestation disrupt natural water distribution and quality.
Conclusion:
Biogeochemical cycles are fundamental to sustaining life and maintaining ecological balance on Earth. They facilitate the continuous recycling of essential elements, support ecosystem stability, regulate climate, and enable biological functions. Understanding these cycles is crucial for addressing environmental challenges and promoting sustainable practices to minimize human impacts on natural processes.
3. How does population ecology explain the dynamics of species populations, including concepts like carrying capacity, r/K selection theory, and population regulation?
Answer:
Population Ecology Defined:
Population ecology is the branch of ecology that studies the size, structure, and dynamics of populations, and how these factors interact with the environment. It seeks to understand the factors that influence population growth, decline, and stability over time.
Key Concepts in Population Ecology:
Population Size and Density:
- Population Size: The number of individuals in a population at a given time.
- Population Density: The number of individuals per unit area or volume, influencing interactions among individuals and resource competition.
Carrying Capacity (K):
- Definition: The maximum number of individuals that an environment can sustainably support without degrading the habitat.
- Determining Factors: Availability of resources (food, water, shelter), environmental conditions, space, and interactions with other species.
- Population Regulation: When a population approaches carrying capacity, factors such as resource limitation, disease, and predation increase mortality rates or decrease birth rates, stabilizing the population.
Population Growth Models:
Exponential Growth (r):
- Description: Occurs when resources are abundant, and populations grow rapidly without constraints.
- Equation: N(t) = N₀e^(rt), where N(t) is population size at time t, N₀ is the initial population size, and r is the intrinsic growth rate.
- Characteristics: J-shaped curve, unsustainable in the long term as it ignores environmental limitations.
Logistic Growth (r/K):
- Description: Accounts for environmental resistance by incorporating carrying capacity.
- Equation: dN/dt = rN(1 – N/K), where N is population size, r is intrinsic growth rate, and K is carrying capacity.
- Characteristics: S-shaped (sigmoidal) curve, indicating slow growth as the population approaches K.
r/K Selection Theory:
r-Selected Species:
- Traits: High reproductive rates, short lifespans, minimal parental care, and high dispersal ability.
- Examples: Insects, rodents, many annual plants.
- Adaptation: Thrive in unstable or unpredictable environments where rapid population growth is advantageous.
K-Selected Species:
- Traits: Lower reproductive rates, longer lifespans, substantial parental care, and lower dispersal ability.
- Examples: Elephants, whales, primates, many large trees.
- Adaptation: Thrive in stable environments near carrying capacity, where competition for resources is intense, and quality over quantity is favored.
Intermediate Strategies:
- Description: Many species exhibit a mix of r and K characteristics, adapting flexibly to varying environmental conditions.
Population Regulation Mechanisms:
Density-Dependent Factors:
- Description: Factors that intensify as population density increases, regulating population size.
- Examples: Competition for resources, predation, disease, and parasitism.
- Impact: Help stabilize populations by limiting growth when density is high.
Density-Independent Factors:
- Description: Factors that affect population size regardless of density.
- Examples: Natural disasters (fires, floods, hurricanes), climate changes, and human activities (pollution, habitat destruction).
- Impact: Can cause sudden population declines but do not provide long-term regulation based on population density.
Life History Strategies:
- Definition: The strategies organisms use to allocate resources to growth, reproduction, and survival.
- Components: Age at first reproduction, number of offspring, investment in offspring, lifespan.
- Trade-Offs: Investments in one aspect (e.g., large number of offspring) may reduce investment in another (e.g., parental care).
Migration and Dispersal:
- Migration: Seasonal movement of populations between breeding and non-breeding areas.
- Dispersal: Movement of individuals away from their natal area to reduce competition and enhance genetic diversity.
- Impact on Population Dynamics: Influences gene flow, population distribution, and can prevent local overpopulation.
Population Genetics:
- Genetic Variation: Essential for populations to adapt to changing environments.
- Factors Influencing Genetic Diversity: Mutation, gene flow, genetic drift, and selection.
- Implications for Population Health: High genetic diversity enhances resilience to diseases and environmental changes, while low diversity can increase vulnerability.
Applications of Population Ecology:
Conservation Biology: Understanding population dynamics aids in developing strategies to protect endangered species and manage wildlife populations.
Resource Management: Insights into population growth help in sustainable harvesting of resources like fisheries and timber.
Epidemiology: Population models can predict the spread of diseases and inform public health interventions.
Agriculture: Managing pest populations through understanding their reproductive and growth patterns.
Conclusion:
Population ecology provides a framework for understanding how species populations grow, interact, and respond to environmental pressures. Concepts like carrying capacity, r/K selection theory, and population regulation are essential for predicting population trends and implementing effective conservation and management strategies. By analyzing the factors that influence population dynamics, ecologists can better comprehend the complexities of ecosystems and the roles different species play within them.
4. What role do keystone species play in maintaining community structure and biodiversity within ecosystems?
Answer:
Keystone Species Defined:
A keystone species is a species that has a disproportionately large impact on its ecological community relative to its abundance. The presence or absence of a keystone species can significantly influence the structure, composition, and function of an ecosystem, often maintaining the balance among various other species.
Roles of Keystone Species:
Regulating Predator-Prey Relationships:
- Top Predators: Keystone predators control the populations of prey species, preventing overgrazing or overpopulation of certain organisms.
- Example: Sea otters in kelp forest ecosystems prey on sea urchins, preventing them from overgrazing kelp and maintaining the structure of the kelp forest, which supports diverse marine life.
Maintaining Species Diversity:
- Preventing Competitive Exclusion: By controlling dominant species, keystone species allow for a greater variety of species to coexist.
- Example: Wolves in Yellowstone National Park regulate elk populations, which in turn allows vegetation like willow and aspen to flourish, supporting a diverse array of other species.
Ecosystem Engineering:
- Modifying Habitat: Some keystone species physically alter their environment, creating habitats for other organisms.
- Example: Beavers build dams that create wetlands, providing habitat for numerous aquatic and terrestrial species.
Facilitating Mutualistic Relationships:
- Enhancing Interactions: Keystone species can promote mutualistic interactions among other species, contributing to ecosystem stability.
- Example: Certain flowering plants rely on specific pollinators; if these pollinators are keystone species, their activity ensures the reproduction of multiple plant species.
Influencing Nutrient Cycling:
- Enhancing Decomposition: Keystone decomposers break down organic matter, recycling nutrients essential for ecosystem productivity.
- Example: Earthworms aerate the soil and decompose organic material, enhancing nutrient availability for plants.
Examples of Keystone Species:
Sea Otters (Enhydra lutris):
- Ecosystem: Kelp forests in the North Pacific.
- Impact: Control sea urchin populations, preventing overgrazing of kelp and maintaining habitat complexity.
African Elephants (Loxodonta africana):
- Ecosystem: African savannas.
- Impact: Modify vegetation by uprooting trees and trampling shrubs, creating open grasslands that support diverse species.
Sea Stars (Pisaster ochraceus):
- Ecosystem: Pacific intertidal zones.
- Impact: Prey on mussels, preventing them from dominating the intertidal community and allowing for greater species diversity.
Wolves (Canis lupus):
- Ecosystem: Various, including North American forests.
- Impact: Regulate herbivore populations, promoting vegetation recovery and enhancing habitat for other species.
Honeybees (Apis mellifera):
- Ecosystem: Global, especially agricultural systems.
- Impact: Pollinate a wide variety of plants, facilitating reproduction and maintaining plant diversity.
Beavers (Castor canadensis):
- Ecosystem: North American wetlands.
- Impact: Build dams that create ponds and wetlands, providing habitats for numerous aquatic and terrestrial species.
Consequences of Keystone Species Loss:
Trophic Cascades: The removal of a keystone species can trigger a cascade of changes throughout the ecosystem, often leading to reduced biodiversity and altered ecosystem functions.
Habitat Degradation: Loss of ecosystem engineers can result in the loss of habitats critical for many species, leading to declines or extinctions.
Reduced Ecosystem Resilience: Ecosystems without keystone species may become less stable and more vulnerable to environmental changes and disturbances.
Importance of Keystone Species in Conservation:
Prioritizing Conservation Efforts: Identifying and protecting keystone species can have widespread positive effects on entire ecosystems.
Ecosystem Restoration: Reintroducing keystone species to degraded ecosystems can help restore balance and biodiversity.
Understanding Ecosystem Dynamics: Studying keystone species provides insights into the complex interactions that sustain ecosystems.
Conclusion:
Keystone species are integral to maintaining the structure and biodiversity of ecological communities. Their unique roles influence a wide range of biological and physical processes, ensuring ecosystem stability and resilience. Protecting keystone species is crucial for conserving biodiversity and sustaining the health of ecosystems, highlighting their paramount importance in ecological research and conservation strategies.
5. How do mutualistic relationships contribute to ecosystem stability, and what are some examples of such interactions?
Answer:
Mutualistic Relationships Defined:
Mutualism is a type of symbiotic relationship where both participating species derive benefits, enhancing their survival, reproduction, or overall fitness. These interactions are integral to ecosystem stability, fostering cooperation, enhancing resource utilization, and promoting biodiversity.
Contribution of Mutualistic Relationships to Ecosystem Stability:
Enhancing Resource Efficiency:
- Complementary Resource Use: Mutualistic partners often utilize different resources or exploit resources more efficiently together than they could individually, leading to increased productivity and resource conservation.
- Example: Mycorrhizal fungi extend the root systems of plants, increasing water and nutrient uptake, while plants provide carbohydrates to the fungi through photosynthesis.
Promoting Biodiversity:
- Facilitating Species Coexistence: Mutualistic interactions can support niche differentiation, allowing multiple species to coexist by reducing direct competition for identical resources.
- Example: Pollinators like bees and a diverse array of flowering plants depend on each other, supporting high plant and insect diversity.
Increasing Ecosystem Resilience:
- Buffering Against Disturbances: Mutualistic networks can provide redundancy, ensuring that ecosystem functions continue even if some interactions are disrupted.
- Example: Multiple pollinators can sustain plant reproduction even if one pollinator species declines, maintaining plant community stability.
Supporting Complex Food Webs:
- Enhancing Trophic Interactions: Mutualistic relationships create intricate connections among species, strengthening the complexity and stability of food webs.
- Example: Coral reefs rely on the mutualism between coral polyps and zooxanthellae algae, supporting diverse marine communities.
Facilitating Nutrient Cycling:
- Efficient Recycling: Mutualists often aid in the decomposition and nutrient recycling processes, enhancing soil fertility and ecosystem productivity.
- Example: Leguminous plants form mutualistic associations with nitrogen-fixing bacteria, enriching soil nitrogen levels for themselves and neighboring plants.
Examples of Mutualistic Interactions:
Pollination Mutualism:
- Bees and Flowers: Bees collect nectar and pollen from flowers for food, inadvertently transferring pollen between flowers, facilitating plant reproduction.
- Impact: Enhances plant genetic diversity, supports agricultural productivity, and maintains ecosystem plant communities.
Mycorrhizal Fungi and Plants:
- Association: Mycorrhizal fungi colonize plant roots, extending their hyphal networks into the soil.
- Benefits to Plants: Improved water and nutrient uptake, particularly phosphorus.
- Benefits to Fungi: Access to carbohydrates produced by plants through photosynthesis.
- Impact: Increases plant growth and soil health, supports diverse plant communities.
Cleaner Fish and Host Fish:
- Example: Cleaner wrasses remove parasites and dead tissue from larger host fish.
- Benefits to Host Fish: Reduced parasite load and improved health.
- Benefits to Cleaner Fish: Access to a reliable food source.
- Impact: Enhances fish health, promotes biodiversity by supporting the survival of multiple host species.
Ants and Acacia Trees:
- Mutualism: Certain ant species inhabit the hollow thorns of Acacia trees, protecting them from herbivores and competing plants.
- Benefits to Acacia Trees: Defense against herbivores and prevention of overgrowth by competing vegetation.
- Benefits to Ants: Shelter and food sources provided by the tree (e.g., nectar, protein-rich Beltian bodies).
- Impact: Maintains Acacia populations and associated biodiversity, supporting a range of other organisms dependent on Acacia habitats.
Lichens (Algae and Fungi):
- Mutualism: Algae perform photosynthesis, providing carbohydrates to the fungal partner, while fungi offer protection and access to nutrients.
- Benefits to Algae: Protection from environmental stressors and enhanced access to water and minerals.
- Benefits to Fungi: Sustained energy supply and access to atmospheric nutrients.
- Impact: Enables colonization of harsh environments, contributes to soil formation, and supports diverse ecosystems by providing foundational species.
Humans and Gut Microbiota:
- Mutualism: Gut bacteria aid in digestion, synthesize essential vitamins, and protect against pathogenic microbes.
- Benefits to Humans: Improved nutrient absorption, immune system support, and overall health.
- Benefits to Bacteria: Stable habitat and access to nutrients from the host’s diet.
- Impact: Maintains human health, influences behavior and metabolism, and contributes to disease prevention.
Coral and Zooxanthellae:
- Mutualism: Zooxanthellae (photosynthetic algae) live within coral tissues, providing energy through photosynthesis.
- Benefits to Corals: Enhanced growth and reef building through the supply of organic compounds.
- Benefits to Zooxanthellae: Protected environment and access to nutrients from coral metabolism.
- Impact: Supports the formation of coral reefs, which are hotspots of marine biodiversity and provide essential ecosystem services like coastal protection and fisheries support.
Termites and Protozoa:
- Mutualism: Termites harbor protozoa in their guts that break down cellulose from wood, providing termites with necessary nutrients.
- Benefits to Termites: Access to energy and nutrients from cellulose digestion.
- Benefits to Protozoa: A stable habitat and continuous supply of cellulose.
- Impact: Facilitates decomposition of wood, contributing to nutrient cycling and soil fertility.
Consequences of Disrupted Mutualistic Relationships:
Reduced Biodiversity: Loss of mutualistic partners can lead to declines in species populations and reduced ecosystem diversity.
Ecosystem Instability: Disruption can impair essential ecosystem functions like pollination, nutrient cycling, and habitat formation, compromising ecosystem health.
Decreased Productivity: Mutualistic interactions often enhance productivity; their loss can result in lower biomass and reduced resource availability.
Conclusion:
Mutualistic relationships are fundamental to ecosystem stability and functionality. By fostering cooperation and enhancing resource utilization, these interactions support biodiversity, increase ecosystem resilience, and maintain essential ecological processes. Protecting and promoting mutualistic partnerships is crucial for sustaining healthy and productive ecosystems, which in turn benefit all forms of life, including humans.
6. What are invasive species, and how do they affect native biodiversity and ecosystem functioning? Provide specific examples.
Answer:
Invasive Species Defined:
Invasive species are non-native organisms introduced to a new environment, where they establish, spread rapidly, and cause harm to native species, ecosystems, human health, or economies. Unlike native species, invasive species often lack natural predators or controls in their introduced habitats, allowing them to proliferate unchecked.
Characteristics of Invasive Species:
High Reproductive Rates: Enable rapid population growth and spread.
Dispersal Ability: Effective means of spreading, such as through seeds, spores, or human-mediated transport.
Generalist Diets: Ability to exploit a wide range of resources reduces competition and enhances survival.
Tolerance to Environmental Conditions: Can thrive in diverse and often disturbed environments.
Effects on Native Biodiversity and Ecosystem Functioning:
Competition for Resources:
- Resource Overuse: Invasive species can outcompete native species for essential resources like food, water, light, and space.
- Example: Zebra Mussels in North American waterways compete with native mussel species for plankton, leading to declines in native populations.
Predation and Herbivory:
- Direct Predation: Invasive predators can decimate native prey populations.
- Herbivory Pressure: Invasive herbivores may overconsume native plants, disrupting plant communities.
- Example: Asian Carp in North American rivers predate on native fish and invertebrates, altering aquatic food webs and reducing biodiversity.
Habitat Alteration:
- Physical Changes: Some invasive species modify habitats, making them unsuitable for native species.
- Example: Kudzu (Pueraria montana) in the southeastern United States grows rapidly, smothering native vegetation and altering forest structure.
Disease Introduction:
- Pathogen Transmission: Invasive species can introduce new diseases or parasites to which native species have no resistance.
- Example: The introduction of the chytrid fungus (Batrachochytrium dendrobatidis) has caused significant declines in amphibian populations worldwide.
Hybridization:
- Genetic Mixing: Invasive species can interbreed with closely related native species, leading to hybrid offspring that may outcompete pure native strains.
- Example: Invasive salmon species hybridizing with native trout can dilute genetic diversity and disrupt local adaptations.
Ecosystem Services Disruption:
- Impact on Services: Invasive species can interfere with ecosystem services such as pollination, water purification, and soil fertility.
- Example: Invasive plants like Japanese knotweed can clog waterways, affecting water flow and increasing flood risks.
Economic Costs:
- Damage to Agriculture and Infrastructure: Invasive species can harm crops, livestock, and damage infrastructure.
- Control Expenses: Significant resources are required to manage and eradicate invasive populations.
- Example: The emerald ash borer (Agrilus planipennis) has devastated ash tree populations in North America, leading to costly tree removals and replacements.
Specific Examples of Invasive Species:
Zebra Mussels (Dreissena polymorpha):
- Origin: Native to freshwater lakes in Eurasia.
- Impact: Outcompete native mussels for plankton, clog water intake pipes, disrupt local aquatic ecosystems, and alter nutrient cycling.
European Starling (Sturnus vulgaris):
- Origin: Native to Europe and Asia.
- Impact: Compete with native bird species for nesting sites and food resources, leading to declines in native bird populations.
Cane Toads (Rhinella marina):
- Origin: Native to Central and South America.
- Impact: Poisonous to native predators, reducing populations of species that attempt to consume them, and compete with native amphibians for resources.
Asian Carp (Hypophthalmichthys molitrix):
- Origin: Native to Asia.
- Impact: Outcompete native fish for planktonic food sources, disrupt aquatic food webs, and alter ecosystem dynamics in North American waterways.
Kudzu (Pueraria montana):
- Origin: Native to Asia.
- Impact: Rapidly overgrows native vegetation, reducing plant diversity, altering soil chemistry, and degrading habitat structures.
Lionfish (Pterois volitans and Pterois miles):
- Origin: Native to the Indo-Pacific region.
- Impact: Predate on native reef fish and invertebrates, disrupting coral reef ecosystems and reducing fish biodiversity.
Brown Tree Snake (Boiga irregularis):
- Origin: Native to Australia and parts of Southeast Asia.
- Impact: Caused the decline or extinction of several native bird and reptile species on Guam, disrupting island ecosystems.
Hydrilla (Hydrilla verticillata):
- Origin: Native to Asia.
- Impact: Forms dense mats in freshwater systems, outcompeting native aquatic plants, altering water flow, and hindering recreational activities.
Emerald Ash Borer (Agrilus planipennis):
- Origin: Native to Asia.
- Impact: Infests and kills ash trees, leading to significant losses in forest and urban tree populations, with extensive economic and ecological consequences.
Red Imported Fire Ant (Solenopsis invicta):
- Origin: Native to South America.
- Impact: Aggressive and venomous, outcompete native ant species, harm wildlife, and pose risks to human health and agriculture.
Management and Control of Invasive Species:
- Prevention:
- Biosecurity Measures: Implementing inspections, quarantine protocols, and public education to prevent the introduction of invasive species.
- Early Detection and Rapid Response:
- Monitoring: Regular surveys and monitoring to detect new invasions early, enabling swift action to eradicate or contain them.
- Mechanical Control:
- Physical Removal: Manual removal, trapping, or mowing of invasive species to reduce their populations.
- Chemical Control:
- Pesticides and Herbicides: Use of chemicals to kill invasive species, though this method can have non-target effects on native organisms.
- Biological Control:
- Introducing Natural Enemies: Introducing predators, parasites, or pathogens that specifically target invasive species, helping to regulate their populations.
- Habitat Restoration:
- Reestablishing Native Species: Restoring native vegetation and ecosystems to outcompete and suppress invasive species.
- Legislation and Policy:
- Regulatory Frameworks: Enforcing laws and regulations that limit the spread of invasive species and support management efforts.
Conclusion:
Invasive species pose significant threats to native biodiversity and ecosystem functioning by disrupting existing ecological balances, outcompeting native species, altering habitats, and impairing ecosystem services. Effective management requires a combination of prevention, early detection, control measures, and habitat restoration. Understanding the mechanisms by which invasive species impact ecosystems is crucial for developing strategies to protect native biodiversity and maintain healthy, resilient ecosystems.
7. How do ecological niches facilitate species coexistence, and what mechanisms prevent competitive exclusion in diverse communities?
Answer:
Ecological Niches Defined:
An ecological niche encompasses the role and position a species has within its ecosystem, including how it obtains resources, interacts with other organisms, and responds to environmental conditions. It represents the total set of environmental conditions and resources required for a species to survive, grow, and reproduce.
Facilitation of Species Coexistence through Ecological Niches:
Niche Differentiation (Resource Partitioning):
- Definition: Species evolve to utilize different resources or the same resources in different ways, reducing direct competition.
- Types:
- Spatial Partitioning: Occupying different physical areas or habitats within the same ecosystem.
- Temporal Partitioning: Utilizing resources at different times (e.g., diurnal vs. nocturnal activity patterns).
- Dietary Partitioning: Consuming different types or sizes of food items.
- Example: Darwin’s finches on the Galápagos Islands have evolved distinct beak shapes to exploit various seed sizes and types, allowing multiple finch species to coexist without direct competition.
Competitive Exclusion Principle:
- Definition: No two species can occupy the exact same niche indefinitely in a stable environment; one species will outcompete and exclude the other.
- Prevention: Niche differentiation and other mechanisms create differences that allow species to coexist by minimizing direct competition.
Mutualistic Interactions:
- Facilitating Coexistence: Mutualistic relationships can reduce competition by providing species with unique benefits that enhance their survival.
- Example: Pollinators and flowering plants rely on each other, ensuring that both can thrive without directly competing for the same resources.
Predation and Herbivory:
- Top-Down Control: Predators regulate the populations of prey species, preventing any one species from becoming overly dominant and allowing others to coexist.
- Example: Wolves in Yellowstone regulate elk populations, preventing overgrazing and allowing vegetation and other species to thrive.
Environmental Heterogeneity:
- Variable Conditions: Diverse and changing environmental conditions create multiple niches, supporting a variety of species with different adaptations.
- Example: Coral reefs offer a multitude of microhabitats, enabling numerous fish species to inhabit different niches within the same ecosystem.
Mechanisms Preventing Competitive Exclusion:
Niche Differentiation:
- Explanation: By specializing in different resources or methods of resource acquisition, species reduce direct competition.
- Outcome: Multiple species can occupy overlapping habitats by utilizing distinct aspects of the environment.
Temporal Segregation:
- Explanation: Species may exploit the same resource at different times to avoid competition.
- Example: Some birds feed during the day while others feed at night, utilizing the same food sources without direct competition.
Spatial Segregation:
- Explanation: Species occupy different physical spaces or microhabitats within the same ecosystem.
- Example: Different fish species may inhabit various depths or sections of a coral reef, reducing overlap in resource use.
Dietary Specialization:
- Explanation: Species develop preferences for different types or sizes of food items.
- Example: Insect species may specialize in feeding on different plant parts, such as leaves, stems, or flowers, minimizing competition.
Adaptive Behaviors:
- Explanation: Behavioral changes can reduce competition by altering how and when species utilize resources.
- Example: Shifts in foraging behavior or habitat use in response to the presence of competitors.
Evolutionary Adaptations:
- Explanation: Over time, species may evolve traits that allow them to exploit different resources or environments.
- Example: Divergent evolution leads to morphological differences, such as beak shapes in finches, enabling resource specialization.
Mutualistic Networks:
- Explanation: Complex mutualistic interactions can create interdependencies that stabilize communities and prevent competitive exclusion.
- Example: Plants and their specific pollinators rely on each other, maintaining species diversity and ecosystem balance.
Environmental Fluctuations:
- Explanation: Dynamic changes in the environment can create temporary niches or reduce competition periodically.
- Example: Seasonal variations can lead to shifts in resource availability, allowing different species to dominate at different times.
Case Studies Illustrating Coexistence Mechanisms:
Darwin’s Finches:
- Niche Differentiation: Various beak shapes adapted to specific seed types, allowing multiple finch species to coexist on the same islands without directly competing for identical food resources.
African Savanna Grazers:
- Spatial and Dietary Partitioning: Different herbivore species (e.g., zebras, wildebeest, gazelles) graze on varying types of vegetation and occupy different spatial niches, reducing competition and supporting high biodiversity.
Coral Reef Fish:
- Microhabitat Utilization: Numerous fish species inhabit distinct niches within the coral reef structure, such as different sections of the reef or specific feeding strategies, facilitating coexistence in a highly competitive environment.
Consequences of Competitive Exclusion:
Species Decline or Extinction: Without mechanisms to prevent competitive exclusion, dominant species can drive others to decline or extinction, reducing biodiversity.
Altered Ecosystem Functioning: Loss of species can disrupt ecological processes, such as pollination, nutrient cycling, and food web dynamics, compromising ecosystem health and resilience.
Conclusion:
Ecological niches are fundamental to the coexistence of species within ecosystems, promoting resource specialization and reducing direct competition. Mechanisms such as niche differentiation, mutualistic interactions, predation, and environmental heterogeneity prevent competitive exclusion, allowing diverse communities to thrive. Understanding these dynamics is essential for conserving biodiversity and maintaining the stability and functionality of ecosystems.
8. What are ecosystem services, how are they categorized, and why are they important for human well-being?
Answer:
Ecosystem Services Defined:
Ecosystem services are the multitude of benefits that humans derive from ecosystems. These services are essential for survival, economic activities, and overall well-being, encompassing both tangible and intangible benefits provided by natural environments.
Categories of Ecosystem Services:
Provisioning Services:
- Definition: Products obtained from ecosystems that directly satisfy human needs.
- Examples:
- Food: Crops, livestock, fish, and wild game.
- Water: Freshwater for drinking, irrigation, and sanitation.
- Raw Materials: Timber, fiber, biofuels, and medicinal resources.
- Genetic Resources: Genetic diversity used for crop improvement and pharmaceutical development.
- Importance: Essential for human sustenance, economic development, and industrial processes.
Regulating Services:
- Definition: Benefits obtained from the regulation of ecosystem processes.
- Examples:
- Climate Regulation: Carbon sequestration by forests and oceans helps mitigate climate change.
- Water Purification: Wetlands and riparian zones filter pollutants, improving water quality.
- Pollination: Insects like bees and butterflies facilitate plant reproduction.
- Pest Control: Predators and parasitoids regulate populations of agricultural pests.
- Flood Regulation: Vegetation and wetlands absorb excess rainfall, reducing flood risks.
- Importance: Maintain environmental balance, protect against natural disasters, and enhance agricultural productivity.
Supporting Services:
- Definition: Fundamental services necessary for the production of all other ecosystem services.
- Examples:
- Nutrient Cycling: Decomposition and mineralization recycle essential nutrients like nitrogen and phosphorus.
- Soil Formation: Weathering of rocks and accumulation of organic matter create fertile soils.
- Primary Production: Photosynthesis by plants and algae forms the base of food webs, providing energy for all trophic levels.
- Habitat Provision: Ecosystems provide habitats for a diverse array of species, supporting biodiversity.
- Importance: Ensure the sustainability of ecosystems by maintaining essential biological and physical processes.
Cultural Services:
- Definition: Non-material benefits people obtain from ecosystems through spiritual enrichment, cognitive development, reflection, recreation, and aesthetic experiences.
- Examples:
- Recreational Activities: Hiking, bird-watching, fishing, and tourism.
- Spiritual and Religious Values: Sacred natural sites and landscapes hold spiritual significance for various cultures.
- Educational and Scientific Research: Natural environments offer opportunities for learning, research, and innovation.
- Aesthetic Appreciation: Scenic landscapes and biodiversity contribute to human happiness, creativity, and cultural identity.
- Cultural Heritage: Ecosystems play a role in traditional practices, folklore, and cultural narratives, preserving community identities.
- Importance: Enhance quality of life, support mental and emotional well-being, and contribute to cultural identity and heritage.
Importance of Ecosystem Services for Human Well-Being:
- Survival and Sustenance:
- Provisioning Services: Directly provide essential resources like food, water, and shelter, ensuring human survival.
- Economic Benefits:
- Resource Extraction: Raw materials and genetic resources are vital for industries, agriculture, and biotechnology.
- Tourism and Recreation: Cultural and recreational services support tourism industries, generating significant economic revenue.
- Health and Medicine:
- Medicinal Resources: Many pharmaceuticals are derived from natural compounds found in plants, animals, and microorganisms.
- Air and Water Quality: Regulating services like water purification and air filtration improve public health by reducing pollutants and disease vectors.
- Environmental Stability:
- Climate Regulation: Ecosystems help mitigate climate change by sequestering carbon dioxide and regulating local climates.
- Disaster Mitigation: Services like flood regulation and coastal protection reduce the impact of natural disasters on human populations.
- Cultural and Spiritual Enrichment:
- Mental and Emotional Health: Natural environments provide spaces for relaxation, reflection, and recreation, contributing to mental well-being.
- Cultural Identity: Ecosystem features are integral to cultural practices, traditions, and community identities, fostering social cohesion.
- Sustainability and Future Generations:
- Resource Renewal: Supporting services ensure the ongoing availability of resources for future generations, promoting sustainability.
- Biodiversity Conservation: Maintaining diverse ecosystems supports resilience against environmental changes and preserves genetic resources for future use.
Challenges in Valuing and Preserving Ecosystem Services:
Economic Valuation:
- Complexity: Quantifying and monetizing ecosystem services is challenging due to their intangible and interdependent nature.
- Underestimation: Often, the true economic value of ecosystem services is underestimated, leading to inadequate protection and investment.
Policy Integration:
- Incorporation into Decision-Making: Integrating ecosystem services into policy frameworks and land-use planning requires comprehensive understanding and collaboration among stakeholders.
Ecosystem Degradation:
- Loss of Services: Deforestation, pollution, climate change, and habitat destruction can degrade or eliminate ecosystem services, adversely affecting human well-being.
Conflicting Interests:
- Resource Use vs. Conservation: Balancing economic development with the conservation of ecosystem services often involves trade-offs and requires sustainable management strategies.
Conclusion:
Ecosystem services are vital for human survival, economic prosperity, health, and cultural well-being. They encompass a wide range of benefits provided by natural ecosystems, categorized into provisioning, regulating, supporting, and cultural services. Recognizing and valuing these services is crucial for informed decision-making, conservation efforts, and sustainable development. Protecting ecosystem services ensures the continued provision of essential resources and environmental regulation, safeguarding human well-being and the health of the planet for future generations.
9. How does ecological succession drive changes in community structure over time, and what are the differences between primary and secondary succession?
Answer:
Ecological Succession Defined:
Ecological succession is the gradual, directional change in the species composition and structure of an ecological community over time. It leads to the establishment of a stable, mature community known as the climax community, which is well-adapted to the prevailing environmental conditions.
Types of Ecological Succession:
Primary Succession:
Definition: Occurs in lifeless areas where no soil initially exists, such as bare rock surfaces, lava flows, or regions exposed by glacial retreat.
Driving Factors:
- Initial Colonization: Pioneer species, typically hardy organisms like lichens and certain mosses, colonize the barren substrate.
- Soil Formation: These pioneers contribute to soil development by breaking down rock through physical and chemical means and accumulating organic matter as they grow and die.
- Environmental Gradients: Gradual changes in moisture, temperature, and nutrient availability facilitate the establishment of successive plant and animal communities.
Typical Stages:
- Pioneer Stage: Dominated by pioneer species that can tolerate harsh conditions and initiate soil formation.
- Intermediate Stages: Grasses, herbs, and shrubs begin to establish as soil depth and quality improve.
- Climax Community: A stable, mature community (e.g., forest) that remains relatively unchanged unless disturbed by external factors.
Examples:
- Lava Flows: After a volcanic eruption, pioneer species colonize the fresh lava, gradually leading to forest development.
- Glacial Retreat Areas: Newly exposed land from receding glaciers undergo primary succession, eventually forming diverse plant and animal communities.
Secondary Succession:
Definition: Occurs in areas where a community previously existed but was removed by a disturbance, leaving the soil intact. Examples include areas after forest fires, agricultural abandonment, or hurricanes.
Driving Factors:
- Disturbance: An event disrupts the existing community but does not eliminate the soil, allowing for quicker recolonization.
- Seed Bank and Residual Organisms: Seeds present in the soil and surviving organisms facilitate rapid re-establishment of plant and animal communities.
- Resource Availability: Existing soil nutrients and structure support faster growth and recovery of species.
Typical Stages:
- Pioneer Stage: Fast-growing, weedy species (e.g., grasses, herbs) quickly colonize the area, stabilizing the soil.
- Intermediate Stages: Shrubs and young trees replace pioneer species as conditions become more favorable.
- Climax Community: A mature forest or other stable community re-establishes, similar to the original pre-disturbance community.
Examples:
- Forest Fires: After a wildfire, grasses and shrubs recolonize, followed by trees as the soil remains fertile and seed sources are nearby.
- Abandoned Farmlands: Fields left fallow undergo secondary succession, progressing from grasslands to shrublands and eventually to forested areas.
Differences Between Primary and Secondary Succession:
Initial Conditions:
- Primary Succession: Begins on barren substrates without soil.
- Secondary Succession: Starts in areas with existing soil and remnants of the previous community.
Pioneer Species:
- Primary Succession: Typically lichens, mosses, and hardy grasses.
- Secondary Succession: Usually fast-growing herbaceous plants and shrubs.
Pace of Succession:
- Primary Succession: Generally slower due to initial soil formation.
- Secondary Succession: Faster as soil and seed banks are already present.
Stages and Complexity:
- Primary Succession: Involves more gradual development of soil and complex communities.
- Secondary Succession: Progresses more rapidly to complex communities, often reaching climax stages quicker.
Factors Influencing Ecological Succession:
Disturbance Frequency and Intensity:
- High Frequency: May prevent the establishment of late-successional species, maintaining early-successional communities.
- Low Frequency: Allows communities to progress to more complex stages.
Species Traits:
- Life History Strategies: Traits such as growth rate, reproductive capacity, and dispersal ability influence species’ roles in succession.
Environmental Conditions:
- Climate, Soil, and Topography: These factors determine which species can establish and thrive during different successional stages.
Biotic Interactions:
- Competition, Predation, and Facilitation: Interactions among species influence community dynamics and succession pathways.
Implications of Ecological Succession:
Biodiversity Enhancement:
- Increase in Species Diversity: Succession often leads to higher biodiversity as more niches become available and different species colonize the area.
Ecosystem Services:
- Improved Services: Mature ecosystems typically provide more ecosystem services, such as carbon sequestration, water regulation, and habitat provision.
Conservation and Restoration:
- Guiding Restoration Efforts: Understanding succession helps in designing effective restoration strategies by facilitating the natural recovery of ecosystems.
Resilience and Stability:
- Ecosystem Resilience: Successional stages contribute to ecosystem resilience, enabling ecosystems to recover from disturbances and maintain stability.
Conclusion:
Ecological succession is a fundamental process that drives changes in community structure and composition over time, leading to the establishment of stable climax communities. Primary and secondary succession differ in their initial conditions and pace, with primary succession occurring on barren substrates and secondary succession following disturbances in established communities. Understanding ecological succession is crucial for managing ecosystems, restoring degraded habitats, and conserving biodiversity, as it provides insights into the natural progression and recovery of ecological communities.
10. In what ways does climate change impact ecosystems and their biodiversity, and how can ecosystems adapt to these changes?
Answer:
Climate Change Defined:
Climate change refers to significant, long-term alterations in global or regional climate patterns, primarily driven by human activities such as the burning of fossil fuels, deforestation, and industrial processes. These changes result in increased concentrations of greenhouse gases like carbon dioxide (CO₂), methane (CH₄), and nitrous oxide (N₂O), leading to global warming and various climatic disruptions.
Impacts of Climate Change on Ecosystems and Biodiversity:
Altered Species Distributions:
- Range Shifts: Many species are moving toward higher latitudes and elevations in response to changing temperature and precipitation patterns.
- Example: Mountain species may shift to higher elevations, risking habitat loss as they run out of available space.
Phenological Changes:
- Timing of Biological Events: Changes in the timing of flowering, breeding, migration, and other life cycle events.
- Example: Earlier flowering of plants may lead to mismatches with pollinator activity, affecting plant reproduction and pollinator survival.
Habitat Loss and Fragmentation:
- Destruction of Ecosystems: Rising temperatures, sea-level rise, and extreme weather events can lead to the loss or fragmentation of critical habitats.
- Example: Coral reefs are experiencing bleaching due to elevated sea temperatures, leading to loss of habitat for numerous marine species.
Ocean Acidification:
- Chemical Changes: Increased CO₂ absorption by oceans lowers pH levels, affecting marine life.
- Impact: Calcifying organisms like corals, mollusks, and some plankton struggle to build shells and skeletons, disrupting marine food webs.
Increased Frequency of Extreme Weather Events:
- Storms, Droughts, and Heatwaves: More frequent and intense weather events can cause immediate and long-term damage to ecosystems.
- Example: Severe droughts can lead to forest die-offs, reducing habitat complexity and biodiversity.
Loss of Biodiversity:
- Extinction Risks: Rapid environmental changes can exceed the adaptive capacity of species, leading to local and global extinctions.
- Example: Polar bears are threatened by the loss of sea ice habitat due to rising temperatures, impacting their survival and the Arctic ecosystem.
Disrupted Ecosystem Services:
- Impact on Services: Climate-induced changes can impair ecosystem services like pollination, water purification, and carbon sequestration.
- Example: Reduced pollinator populations affect crop yields and food security, while diminished forests limit carbon storage capabilities.
Invasive Species Proliferation:
- New Opportunities: Climate change can create favorable conditions for invasive species to establish and spread, further threatening native biodiversity.
- Example: Warmer temperatures may allow tropical invasive species to thrive in temperate regions, outcompeting native flora and fauna.
Adaptation Strategies for Ecosystems:
Migration and Range Shifts:
- Natural Movement: Species may naturally migrate to more suitable habitats as conditions change.
- Assisted Migration: Human intervention may be necessary to help species reach new habitats, especially those unable to move quickly enough.
Genetic Adaptation:
- Evolutionary Changes: Populations can evolve traits that enhance their survival under new environmental conditions.
- Genetic Diversity: Maintaining high genetic diversity within populations increases the likelihood of adaptive responses.
Habitat Restoration and Connectivity:
- Enhancing Resilience: Restoring degraded habitats and creating corridors facilitate species movement and gene flow, supporting adaptation.
- Example: Establishing wildlife corridors between fragmented forests allows species to migrate in response to climate shifts.
Ecosystem-Based Management:
- Integrated Approaches: Managing ecosystems holistically to maintain their integrity and functionality, enhancing their capacity to adapt to climate change.
- Example: Protecting keystone species and maintaining ecosystem services like wetlands for flood regulation.
Conservation of Climate Refugia:
- Protected Areas: Identifying and preserving areas less affected by climate change where species can survive and adapt.
- Example: Mountain tops, deep forests, and areas with microclimates can serve as refugia for vulnerable species.
Assisted Evolution and Genetic Engineering:
- Human Intervention: Techniques like selective breeding or genetic modification can enhance species’ resilience to climate stressors.
- Example: Developing drought-resistant crop varieties to sustain agriculture under changing precipitation patterns.
Policy and Governance:
- Climate Action: Implementing policies to mitigate climate change by reducing greenhouse gas emissions and promoting sustainable practices.
- Adaptive Management: Developing flexible management strategies that can adjust to new information and changing conditions.
Public Awareness and Education:
- Engaging Communities: Educating the public about the impacts of climate change and involving them in conservation efforts enhances collective resilience.
- Example: Community-led restoration projects and citizen science initiatives contribute to ecosystem monitoring and restoration.
Case Studies Illustrating Climate Change Impacts and Adaptation:
Coral Reefs:
- Impact: Increased sea temperatures cause coral bleaching, reducing biodiversity and ecosystem services.
- Adaptation: Restoration projects using resilient coral strains and establishing marine protected areas to enhance reef recovery.
Polar Regions:
- Impact: Melting ice habitats threaten species like polar bears and seals.
- Adaptation: Protecting critical habitats, reducing human disturbances, and international cooperation on conservation efforts.
Montane Ecosystems:
- Impact: Species are forced to move to higher elevations, risking habitat loss as they reach the mountain peaks.
- Adaptation: Creating corridors and protected areas to facilitate species migration and preserve diverse elevational habitats.
Conclusion:
Climate change profoundly affects ecosystems and biodiversity by altering species distributions, disrupting phenological events, degrading habitats, and increasing extinction risks. Adaptation strategies, including facilitating species migration, enhancing genetic diversity, restoring habitats, and implementing ecosystem-based management, are essential for sustaining ecosystem resilience and functionality. Proactive conservation efforts and integrated policies are crucial to mitigate the adverse impacts of climate change, preserving biodiversity and the ecosystem services vital for human well-being.
11. How do predators influence the structure and function of ecosystems through trophic cascades?
Answer:
Predators and Trophic Cascades Defined:
Predators: Organisms that hunt, kill, and consume other organisms (prey) for sustenance.
Trophic Cascade: A series of indirect effects that predators have on lower trophic levels, influencing the entire ecosystem’s structure and function.
Mechanisms of Trophic Cascades:
Top-Down Control:
- Direct Predation: Predators directly reduce the populations of their prey.
- Indirect Effects: Reduction in prey populations can lead to increased populations of organisms that the prey species would typically consume, altering the ecosystem dynamics.
Bottom-Up Control:
- Resource Availability: Primary producers (plants and algae) provide the energy base for the ecosystem. Changes in producer populations can influence higher trophic levels.
- Interaction with Trophic Cascades: Both top-down and bottom-up controls interact to shape ecosystem structure.
Examples of Trophic Cascades:
Wolves in Yellowstone National Park:
- Initial Impact: Reintroduction of wolves led to a decline in elk populations.
- Indirect Effects: Reduced grazing pressure by elk allowed willow and aspen trees to recover.
- Further Impact: Increased vegetation supported beaver populations, which built dams that created wetlands, enhancing biodiversity and altering hydrological processes.
- Ecosystem Functioning: Enhanced habitat complexity improved water quality and supported a wider range of species, including birds, amphibians, and fish.
Sea Otters in Kelp Forests:
- Initial Impact: Sea otters prey on sea urchins, controlling their populations.
- Indirect Effects: Reduction in sea urchins prevents overgrazing of kelp, allowing kelp forests to thrive.
- Further Impact: Healthy kelp forests provide habitat for numerous marine species, enhance carbon sequestration, and stabilize coastal ecosystems.
- Ecosystem Functioning: Maintains high biodiversity, supports fisheries, and protects shorelines from erosion.
Top Predators in African Savannas:
- Example: Lions regulate herbivore populations (e.g., zebras, wildebeest).
- Indirect Effects: Controlled herbivore numbers prevent overgrazing, allowing grasslands to remain healthy and support a variety of plant and animal species.
- Ecosystem Functioning: Promotes plant diversity, maintains soil health, and supports scavengers and other secondary consumers.
Tuna and Shark Dynamics in Marine Ecosystems:
- Initial Impact: Declines in shark populations can lead to increased numbers of mid-level predators like tuna.
- Indirect Effects: Elevated tuna populations may overconsume smaller fish and invertebrates, reducing their abundance.
- Ecosystem Functioning: Disruption of fish populations can affect coral reef health, algal growth, and overall marine biodiversity.
Consequences of Altered Predation:
Biodiversity Loss:
- Species Declines: Removal or reduction of predators can lead to overpopulation of certain species, causing imbalances and potential extinction of others.
Habitat Degradation:
- Overgrazing: Excessive herbivore populations can degrade vegetation, leading to soil erosion, reduced plant diversity, and loss of habitat for other species.
Altered Nutrient Cycling:
- Impact on Decomposition: Changes in species populations can influence the rate and efficiency of nutrient cycling processes, affecting soil fertility and ecosystem productivity.
Reduced Ecosystem Resilience:
- Vulnerability to Disturbances: Ecosystems without balanced predation are less resilient to environmental changes and disturbances, making them more susceptible to collapse.
Importance of Predators in Ecosystem Management:
Maintaining Balance: Predators help regulate prey populations, preventing any single species from dominating and maintaining ecological balance.
Enhancing Biodiversity: By controlling dominant species, predators allow for greater species diversity and complexity within ecosystems.
Promoting Healthy Ecosystems: Predators contribute to the overall health and functioning of ecosystems by influencing behavior, distribution, and population dynamics of other species.
Challenges in Understanding and Managing Trophic Cascades:
Complex Interactions: Ecosystems involve intricate food webs with multiple predator-prey relationships, making it challenging to predict the outcomes of altering predator populations.
Human Impacts: Overfishing, habitat destruction, and pollution can disrupt trophic cascades, complicating conservation and management efforts.
Delayed Effects: Trophic cascades can take time to manifest, requiring long-term monitoring and adaptive management strategies.
Conclusion:
Predators play a crucial role in shaping the structure and function of ecosystems through trophic cascades. By regulating prey populations, they maintain ecological balance, promote biodiversity, and enhance ecosystem resilience. Understanding the dynamics of trophic cascades is essential for effective ecosystem management and conservation efforts, ensuring the sustainability and health of natural environments.
12. What strategies can be employed in conservation biology to protect and restore ecosystems and their biodiversity?
Answer:
Conservation Biology Defined:
Conservation biology is a multidisciplinary field focused on understanding and mitigating the loss of biodiversity, protecting ecosystems, and ensuring the sustainable management of natural resources. It employs scientific principles to develop strategies aimed at preserving species, habitats, and ecological processes.
Strategies in Conservation Biology:
Protected Areas and Reserves:
- Establishment of Protected Zones: Designating national parks, wildlife reserves, and marine protected areas to safeguard critical habitats and species.
- Impact: Prevent habitat destruction, reduce human disturbances, and provide refuges for endangered species.
- Example: The creation of the Great Barrier Reef Marine Park protects diverse marine life and supports sustainable tourism.
Habitat Restoration and Rehabilitation:
- Ecosystem Restoration: Rehabilitating degraded or destroyed ecosystems to restore their structure, function, and biodiversity.
- Techniques: Reforestation, wetland restoration, removal of invasive species, and reintroduction of native species.
- Example: Restoring wetlands in Louisiana to support migratory birds and improve water quality.
Species Reintroduction and Recovery Programs:
- Reintroduction: Bringing back species that have been extirpated from certain areas to restore ecological balance.
- Recovery Programs: Targeted efforts to increase populations of endangered or threatened species.
- Example: The reintroduction of gray wolves to Yellowstone National Park has restored trophic cascades and ecosystem health.
Invasive Species Management:
- Control and Eradication: Implementing measures to control or eliminate invasive species that threaten native biodiversity.
- Preventative Actions: Biosecurity measures to prevent the introduction and spread of invasive species.
- Example: Removing invasive plants like kudzu to allow native vegetation to recover and support local wildlife.
Ex Situ Conservation:
- Captive Breeding: Breeding endangered species in controlled environments like zoos and botanical gardens.
- Seed Banks: Preserving seeds of plant species for future restoration and reintroduction.
- Genetic Repositories: Maintaining genetic diversity through DNA banks and biobanks.
- Example: The Svalbard Global Seed Vault stores seeds from around the world to safeguard against catastrophic loss.
Sustainable Resource Management:
- Fisheries Management: Implementing quotas, marine protected areas, and sustainable fishing practices to prevent overfishing.
- Forestry Practices: Promoting selective logging, reforestation, and sustainable timber harvesting to maintain forest ecosystems.
- Agricultural Sustainability: Encouraging practices like crop rotation, organic farming, and integrated pest management to preserve soil health and biodiversity.
- Example: The use of sustainable forestry certifications (e.g., FSC) ensures that timber is harvested responsibly, protecting forest ecosystems.
Climate Change Mitigation and Adaptation:
- Reducing Emissions: Implementing policies and practices to lower greenhouse gas emissions, thereby mitigating climate change impacts.
- Enhancing Resilience: Developing strategies to help ecosystems and species adapt to changing climatic conditions.
- Example: Coastal mangrove restoration enhances carbon sequestration, provides storm protection, and supports marine biodiversity.
Community-Based Conservation:
- Engaging Local Communities: Involving indigenous peoples and local stakeholders in conservation efforts ensures that strategies are culturally appropriate and gain community support.
- Empowering Communities: Providing education, resources, and incentives for sustainable practices.
- Example: Community-managed forests in Nepal balance timber production with conservation, benefiting both people and biodiversity.
Legal and Policy Frameworks:
- Enforcing Laws: Implementing and enforcing environmental protection laws and regulations to safeguard habitats and species.
- International Agreements: Participating in global treaties like the Convention on Biological Diversity (CBD) to promote international cooperation.
- Example: The Endangered Species Act (ESA) in the United States provides legal protection for threatened and endangered species.
Biodiversity Monitoring and Research:
- Data Collection: Conducting surveys, tracking populations, and monitoring ecosystem health to inform conservation strategies.
- Scientific Research: Studying ecological processes, species interactions, and the impacts of human activities to develop evidence-based conservation approaches.
- Example: Long-term monitoring of bird populations helps detect declines early, allowing for timely conservation interventions.
Restoration of Ecosystem Functions:
- Functional Restoration: Reestablishing key ecological processes like pollination, nutrient cycling, and natural fire regimes to enhance ecosystem health.
- Example: Reintroducing fire in fire-dependent ecosystems like prairies to maintain plant diversity and prevent woody plant encroachment.
Public Awareness and Education:
- Raising Awareness: Educating the public about the importance of biodiversity and the threats it faces encourages support for conservation initiatives.
- Promoting Stewardship: Fostering a sense of responsibility and encouraging individuals to participate in conservation activities.
- Example: Campaigns like Earth Hour inspire global participation in environmental protection efforts.
Challenges in Conservation Biology:
- Funding and Resources:
- Limited Funding: Adequate financial resources are often scarce, hindering the implementation of comprehensive conservation programs.
- Political and Economic Barriers:
- Policy Inconsistencies: Political instability, lack of enforcement, and conflicting economic interests can impede conservation efforts.
- Climate Change:
- Dynamic Conditions: Rapid environmental changes make it difficult to predict and respond to conservation needs effectively.
- Invasive Species:
- Persistent Threats: Controlling invasive species requires sustained efforts and can be resource-intensive.
- Human-Wildlife Conflicts:
- Competing Interests: Balancing human development and wildlife conservation can lead to conflicts and resistance from local communities.
Conclusion:
Conservation biology employs a multifaceted approach to protect and restore ecosystems and their biodiversity. Strategies range from establishing protected areas and restoring habitats to managing invasive species and engaging local communities. Overcoming challenges such as limited funding, political barriers, and climate change is essential for the success of conservation efforts. By integrating scientific research, sustainable practices, and community involvement, conservation biology aims to preserve the intricate web of life that sustains ecosystems and, ultimately, human well-being.
12. How do cell adhesion molecules (CAMs) influence cell signaling and behavior, and what are the implications for tissue development and disease?
Answer:
Cell Adhesion Molecules (CAMs) Defined:
Cell adhesion molecules (CAMs) are proteins located on the cell surface that mediate the binding between cells and between cells and the extracellular matrix (ECM). They play crucial roles in maintaining tissue structure, facilitating communication, and regulating various cellular processes.
Types of CAMs:
Cadherins:
- Characteristics: Calcium-dependent transmembrane proteins that mediate homophilic (same-type) cell-cell adhesion.
- Examples: E-cadherin (epithelial cells), N-cadherin (neuronal and mesenchymal cells).
Integrins:
- Characteristics: Heterodimeric transmembrane receptors composed of α and β subunits that bind to ECM proteins and mediate cell-ECM adhesion.
- Functions: Facilitate cell migration, signal transduction, and maintenance of cell shape.
Selectins:
- Characteristics: Carbohydrate-binding transmembrane proteins involved in transient cell-cell adhesion.
- Examples: E-selectin, P-selectin, L-selectin.
Immunoglobulin Superfamily CAMs (Ig-CAMs):
- Characteristics: Transmembrane proteins with immunoglobulin-like domains that mediate both cell-cell and cell-ECM interactions.
- Examples: ICAMs (Intercellular Adhesion Molecules), VCAMs (Vascular Cell Adhesion Molecules), NCAMs (Neural Cell Adhesion Molecules).
Influence of CAMs on Cell Signaling and Behavior:
Signal Transduction:
- Bidirectional Signaling: CAMs can transmit signals from the extracellular environment to the cell interior (outside-in signaling) and from the cell to the extracellular environment (inside-out signaling).
- Examples: Integrin binding to ECM proteins activates intracellular pathways like focal adhesion kinase (FAK) and the MAPK/ERK pathway, influencing cell survival, proliferation, and differentiation.
Regulation of Cell Growth and Differentiation:
- E-cadherin and β-catenin: E-cadherin-mediated adhesion interacts with β-catenin, regulating gene expression related to cell proliferation and differentiation.
- Impact: Loss of E-cadherin is associated with increased cell proliferation and metastasis in cancer.
Cell Migration and Movement:
- Integrins and Actin Cytoskeleton: Integrins link the ECM to the actin cytoskeleton, facilitating cell movement through processes like lamellipodia and filopodia extension.
- Impact: Essential for wound healing, immune cell trafficking, and embryonic development.
Tissue Organization and Morphogenesis:
- Cadherins in Cell Sorting: Differential expression of cadherins facilitates cell sorting during embryonic development, ensuring proper tissue and organ formation.
- Example: N-cadherin and E-cadherin guide the formation of neural and epithelial tissues, respectively.
Maintaining Tissue Integrity:
- Adherens Junctions and Desmosomes: CAMs like cadherins form adherens junctions and desmosomes, providing mechanical strength and maintaining cell-cell adhesion in tissues.
- Impact: Prevent tissue disintegration under mechanical stress, such as in skin and cardiac muscle.
Immune Responses:
- ICAMs and VCAMs: Facilitate the adhesion and transmigration of leukocytes across endothelial barriers during immune surveillance and inflammation.
- Impact: Enable immune cells to reach sites of infection or injury, playing a role in immune defense mechanisms.
Implications for Tissue Development and Disease:
Tissue Development (Morphogenesis):
- Cell Adhesion and Communication: CAMs coordinate cell positioning, movement, and differentiation during embryonic development, ensuring the formation of complex tissues and organs.
- Example: Neural cell adhesion molecules (NCAMs) guide the growth of neurons and the formation of neural networks.
Cancer Progression and Metastasis:
- Loss of CAM Function: Downregulation or mutation of CAMs like E-cadherin disrupts cell-cell adhesion, facilitating cancer cell detachment, invasion, and metastasis.
- Impact: Enhances tumor aggressiveness and the spread of cancer to distant sites.
Inflammatory Diseases:
- Aberrant CAM Expression: Overexpression or dysfunction of CAMs like ICAMs and VCAMs can lead to excessive leukocyte adhesion and infiltration, contributing to chronic inflammation and autoimmune disorders.
- Example: Elevated ICAM-1 levels are associated with rheumatoid arthritis and multiple sclerosis.
Genetic Disorders:
- Mutations in CAM Genes: Genetic defects in CAMs can result in developmental abnormalities, impaired tissue integrity, and increased susceptibility to diseases.
- Example: Mutations in the CDH1 gene encoding E-cadherin are linked to hereditary diffuse gastric cancer and lobular breast cancer.
Wound Healing and Tissue Repair:
- CAM-Mediated Cell Migration: CAMs facilitate the migration of cells like fibroblasts and epithelial cells to wound sites, promoting tissue repair and regeneration.
- Impact: Efficient wound healing relies on proper CAM function to re-establish tissue integrity and structure.
Neurological Disorders:
- CAMs in Neural Connectivity: Disruptions in CAM-mediated interactions can impair neural network formation, leading to neurological conditions.
- Example: Altered NCAM function is implicated in neurodevelopmental disorders like schizophrenia and autism.
Therapeutic Applications:
Targeting CAMs in Cancer Therapy:
- Inhibition of Metastasis: Developing drugs that restore or enhance CAM function can prevent cancer cell detachment and metastasis.
- Example: Monoclonal antibodies targeting E-cadherin to inhibit tumor spread.
Anti-Inflammatory Treatments:
- Blocking CAM Interactions: Using molecules that inhibit CAM-mediated leukocyte adhesion can reduce excessive inflammation.
- Example: Natalizumab, an antibody against α4-integrin, used in treating multiple sclerosis by preventing immune cell migration into the brain.
Enhancing Wound Healing:
- Promoting CAM Expression: Therapies that boost CAM function can improve cell adhesion and migration, accelerating tissue repair.
- Example: Growth factors that upregulate E-cadherin expression in epithelial cells.
Conclusion:
Cell adhesion molecules are pivotal in regulating cell signaling, behavior, and interactions within tissues. They influence processes such as cell growth, migration, differentiation, and tissue integrity, playing essential roles in development and maintaining healthy tissues. Dysfunctions or alterations in CAMs are implicated in various diseases, including cancer, inflammatory disorders, and genetic conditions. Understanding the functions and mechanisms of CAMs offers valuable insights for developing therapeutic strategies aimed at treating diseases and promoting tissue health and regeneration.