DNA Technology and Genetic Engineering
DNA technology lies at the forefront of modern biology, revolutionizing our understanding of genetic material and enabling powerful applications across healthcare, agriculture, and research. To appreciate its impact, it is essential to build a solid foundation in the broader landscape of science and specifically biology. This includes insights from related disciplines like cell biology, where studies on cell communication, cell cycle, cell development, and cell physiology help uncover the cellular context where DNA functions.
Understanding DNA’s structure and function is closely linked to topics such as cell structure and the ecological consequences of genetic variation, as explored in ecology. At a broader evolutionary scale, evolutionary biology and the study of molecular evolution provide insight into how DNA changes over time, shaping species and populations.
To grasp the full scope of DNA technology, learners must also delve into genetics and its many branches. This includes foundational areas like genomics, Mendelian genetics, and advanced fields such as molecular genetics. A deeper appreciation emerges when one examines how applications of genetics in medicines are reshaping diagnostic and therapeutic strategies.
Central to DNA technology is the manipulation of genetic material. This includes processes like transcription and translation, studied under gene expression, and phenomena such as genetic mutation. These are crucial for understanding molecular basis of inheritance and for designing genetic interventions. Mastery of molecular techniques in research further equips students with the tools to analyze, amplify, or edit DNA sequences with precision.
Complementary areas like protein synthesis help connect DNA changes to phenotypic outcomes, while studies in population genetics and quantitative genetics reveal how DNA influences traits at the group level. Taken together, this knowledge empowers students to explore how DNA technology is applied in diverse sectors—from gene therapy and forensic science to agriculture and synthetic biology—shaping the future of science and society.
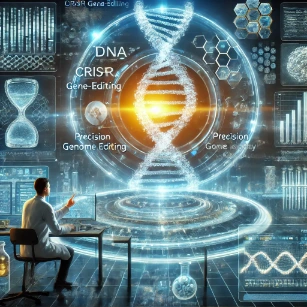
Table of Contents
Tools of DNA Technology
Polymerase Chain Reaction (PCR):
- What It Is:
- PCR is a technique used to amplify specific DNA sequences, producing millions to billions of copies from a tiny initial sample.
- How It Works:
- Involves repeated cycles of denaturation (separating DNA strands), annealing (binding primers to the target sequence), and extension (synthesizing new DNA using a heat-stable DNA polymerase like Taq polymerase).
- Applications:
- Diagnostic tests (e.g., COVID-19 testing).
- Forensic science (DNA fingerprinting).
- Research (amplifying genes for study or cloning).
- What It Is:
Gel Electrophoresis:
- What It Is:
- A method to separate DNA, RNA, or protein fragments based on size using an electric field and a gel matrix.
- How It Works:
- DNA fragments move through an agarose gel towards the positive electrode. Smaller fragments migrate faster, allowing separation.
- Applications:
- Visualizing PCR products.
- Analyzing genetic material for mutations or variations.
- DNA fingerprinting and molecular diagnostics.
- What It Is:
CRISPR-Cas9 Technology:
- What It Is:
- A powerful genome-editing tool derived from bacterial immune systems.
- How It Works:
- The Cas9 enzyme is guided by an RNA sequence (gRNA) to a specific DNA target. Cas9 then cuts the DNA, enabling the insertion, deletion, or alteration of genetic material.
- Applications:
- Editing disease-causing mutations (e.g., in sickle cell disease).
- Developing transgenic crops with improved traits.
- Creating model organisms for research.
- What It Is:
Techniques in Genetic Engineering
Cloning:
- What It Is:
- The process of creating genetically identical copies of organisms, cells, or DNA.
- Types:
- Reproductive Cloning: Produces entire organisms, such as Dolly the sheep.
- Therapeutic Cloning: Generates tissues or organs for medical purposes.
- Applications:
- Studying developmental biology.
- Producing genetically identical crops and livestock.
- Generating cells for regenerative medicine.
- What It Is:
Recombinant DNA Technology:
- What It Is:
- Combines DNA from two or more sources into a single molecule, often using a vector like a plasmid.
- How It Works:
- DNA from the donor organism is inserted into a vector using restriction enzymes and ligases. The vector is introduced into host cells, which replicate and express the recombinant DNA.
- Applications:
- Producing insulin, growth hormones, and vaccines.
- Creating genetically modified organisms (GMOs) with desirable traits.
- Developing DNA-based diagnostic tools.
- What It Is:
Gene Therapy:
- What It Is:
- Involves the introduction, removal, or alteration of genes within a person’s cells to treat or prevent diseases.
- Techniques:
- Viral vectors deliver the therapeutic gene to the target cells.
- Non-viral methods, such as nanoparticles, are used in some cases.
- Applications:
- Treating inherited disorders like cystic fibrosis and Duchenne muscular dystrophy.
- Developing CAR-T cell therapies for cancer.
- Addressing vision loss in inherited retinal diseases.
- What It Is:
Applications of DNA Technology and Genetic Engineering
-
Medicine:
- Diagnostics:
- PCR-based tests detect infectious diseases (e.g., HIV, tuberculosis) and genetic disorders.
- Therapeutics:
- Gene editing corrects mutations causing diseases such as beta-thalassemia.
- Recombinant DNA technology is used to produce therapeutic proteins, such as insulin and monoclonal antibodies.
- Personalized Medicine:
- Pharmacogenomics tailors drug therapies based on genetic profiles.
- Vaccine Development:
- DNA and RNA vaccines (e.g., for COVID-19) are faster and safer to produce.
- Diagnostics:
-
Agriculture:
- Genetically Modified Crops:
- Enhancements include resistance to pests, herbicides, and diseases.
- Examples: Bt cotton, Golden rice enriched with vitamin A, and drought-tolerant maize.
- Livestock Engineering:
- Producing animals with increased growth rates, disease resistance, or enhanced nutritional profiles.
- Food Production:
- Biotechnological processes improve yield, shelf life, and nutrient content in food products.
- Genetically Modified Crops:
-
Environmental Science:
- Bioremediation:
- Engineered microbes clean up oil spills, degrade plastics, and detoxify pollutants.
- Biofuels:
- Genetically modified organisms (GMOs) optimize the production of ethanol and biodiesel from renewable resources.
- Conservation Biology:
- Genetic engineering revives extinct or endangered species and improves genetic diversity in threatened populations.
- Bioremediation:
Why Study DNA Technology
Advancements in Genetic Engineering
DNA technology allows scientists to manipulate genes for research, medicine, and industry. Students learn about tools like recombinant DNA, CRISPR, and cloning. These techniques enable the modification of organisms for specific purposes. Mastering them opens doors to careers in biotechnology and genetic research.
Applications in Medicine
DNA technology is used in diagnosing genetic diseases, developing vaccines, and producing therapeutic proteins. Students explore how gene therapy and molecular diagnostics work. These innovations improve healthcare and save lives. Knowledge in this field prepares students for impactful work in medical science.
Forensic and Legal Applications
DNA fingerprinting and profiling are vital in forensic investigations and paternity testing. Students learn how genetic material can provide evidence in legal cases. This intersection of science and law is growing in importance. It opens pathways in criminal justice, law enforcement, and forensic biology.
Agricultural and Environmental Uses
DNA technology improves crop yield, pest resistance, and environmental sustainability. Students learn how genetically modified organisms (GMOs) are created and regulated. These applications address global challenges in food security and climate change. It equips students to contribute to sustainable agriculture.
Ethical and Regulatory Considerations
With powerful tools come ethical responsibilities in using DNA technology. Students examine issues such as gene editing, cloning, and privacy concerns. Understanding these implications fosters responsible scientific practice. It encourages thoughtful engagement with emerging technologies and public policy.
DNA Technology and Genetic Engineering: Conclusion
DNA technology and genetic engineering have revolutionized science, offering tools and techniques that extend far beyond traditional biology. From curing diseases and improving agricultural productivity to addressing environmental challenges, these innovations demonstrate the immense potential of genetic science. Ongoing advancements in tools like CRISPR, synthetic biology, and genomic sequencing promise even greater possibilities, shaping a future where precision and innovation address some of humanity’s most pressing problems.
DNA Technology and Genetic Engineering: Review Questions and Answers
Question 1
Explain the term “recombinant DNA” and discuss one common application of this technique in biotechnology.
Answer: Recombinant DNA involves joining DNA molecules from two or more different sources to form a new genetic combination. A common application is the production of human insulin by inserting the human insulin gene into bacterial plasmids. The modified bacteria then synthesize insulin, which can be purified for medical use. This process is faster, more efficient, and more ethically viable than extracting insulin from animal pancreases.
Question 2
How does the polymerase chain reaction (PCR) amplify DNA, and why is it so crucial in modern molecular genetics?
Answer: PCR amplifies DNA by repeatedly cycling through denaturation, annealing, and extension steps:
- Denaturation: Heating separates the double-stranded DNA into single strands.
- Annealing: Primers bind (anneal) to their complementary sequences on each DNA strand when the temperature is lowered.
- Extension: DNA polymerase extends the primers, synthesizing new DNA strands.
Repeated cycles exponentially increase the number of DNA copies. PCR is crucial for diagnostics (e.g., detecting pathogens), forensics (e.g., amplifying trace DNA), research (e.g., cloning genes), and many other areas that require large quantities of specific DNA segments.
Question 3
What is the principle behind CRISPR-Cas9 gene editing, and what makes it more efficient than older gene-editing methods?
Answer: CRISPR-Cas9 relies on a guide RNA (gRNA) that directs the Cas9 nuclease to a specific DNA sequence where Cas9 can make a targeted cut. Afterward, natural DNA repair processes either introduce small mutations (non-homologous end joining) or insert a new gene sequence (homologous recombination). CRISPR-Cas9 is more efficient because:
- It has high specificity due to the custom-designed guide RNA.
- It is versatile and easier to engineer than older methods like zinc-finger nucleases or TALENs.
- It can be adapted for multiple simultaneous edits, speeding up experimental and therapeutic applications.
Question 4
How do restriction enzymes assist in DNA cloning, and what is the significance of ‘sticky ends’?
Answer: Restriction enzymes cut DNA at specific recognition sites, generating fragments. Some restriction enzymes produce “sticky ends,” which are single-stranded overhangs. When two DNA fragments are cut with the same enzyme, their sticky ends are complementary, enabling them to base-pair and be joined by DNA ligase. This property is crucial for cloning: scientists can insert a gene of interest into a plasmid or vector precisely, ensuring the recombinant molecule can be stably introduced into host cells.
Question 5
Outline the key steps in gene cloning using a bacterial plasmid vector.
Answer:
- Isolation of DNA: Extract and isolate both the target gene DNA and the plasmid DNA.
- Cutting DNA: Use the same restriction enzyme to cut the plasmid vector and the DNA fragment of interest, creating compatible sticky ends.
- Ligation: Mix the cut plasmid and DNA fragment with DNA ligase. The sticky ends anneal, and ligase seals the backbone.
- Transformation: Introduce the recombinant plasmid into competent bacterial cells (e.g., via heat shock).
- Selection: Plate the bacteria on selective media (often containing an antibiotic). Only bacteria that have taken up the plasmid survive.
- Screening and Verification: Verify successful cloning through techniques like PCR, restriction digestion analysis, or DNA sequencing.
Question 6
What is a “DNA library,” and how does a genomic library differ from a cDNA library?
Answer: A DNA library is a collection of DNA fragments stored in host cells or vectors.
- Genomic Library: Contains fragments representing the entire genomic DNA of an organism, including coding and non-coding regions (introns, regulatory sequences).
- cDNA Library: Made from messenger RNA (mRNA) via reverse transcription, so it primarily includes only the expressed genes (exons) of an organism’s cells at the time of mRNA extraction.
Genomic libraries are useful for studying regulatory elements and entire gene structures, whereas cDNA libraries are preferred for analyzing expressed proteins and functional genes.
Question 7
Describe one way DNA technology can aid in the development of genetically modified organisms (GMOs) for agriculture.
Answer: One common approach is transferring pest-resistant or herbicide-tolerant genes into crop plants. For example, a bacterial gene (Bt toxin) can be inserted into corn or cotton, allowing the plant to produce a protein lethal to certain insects but harmless to humans. This genetic modification reduces the need for chemical pesticides, lowers production costs, and can increase crop yields.
Question 8
What is gene therapy, and how might vectors like viruses be employed in this process?
Answer: Gene therapy aims to treat or prevent diseases by introducing, removing, or modifying genes within a patient’s cells. Viruses, engineered to be harmless, can act as vectors that carry and deliver therapeutic genes into human cells. Once inside, these vectors incorporate the healthy gene or help express it, potentially correcting the underlying genetic defect causing the disease. Safety and specificity remain critical concerns in designing these viral vectors.
Question 9
How can DNA microarrays be used to compare gene expression between different cell types or conditions?
Answer: DNA microarrays (gene chips) consist of thousands of immobilized DNA probes. When fluorescently labeled cDNA samples from different cells or conditions are applied, they hybridize to complementary probes on the array. By comparing the fluorescence intensity, researchers see which genes are up or downregulated. This technology allows large-scale analysis of gene expression patterns, helping identify disease markers and understand how cells respond to various stimuli or treatments.
Question 10
Why is ethical and regulatory oversight important in DNA technology, especially concerning gene editing and GMOs?
Answer: DNA technology has vast potential benefits, such as curing diseases and improving food security. However, it also raises concerns:
- Safety and Unintended Consequences: Edited genes might have off-target effects or ecological impacts if released into the environment.
- Informed Consent and Equity: Human gene therapy and edited organisms must be developed responsibly, ensuring fair access and adherence to ethical standards.
- Long-Term Effects: Germline modifications can be passed on to future generations, meaning decisions made today could have irreversible impacts.
Regulatory frameworks help balance scientific innovation with public welfare and environmental protection.
DNA Technology and Genetic Engineering: Thought-Provoking Questions
Below are 12 thought-provoking questions and in-depth answers inspired by the core themes typically covered in a discussion of DNA technology—from the fundamentals of recombinant DNA to emerging applications like CRISPR and gene therapy. These questions aim to prompt deeper consideration of both scientific and societal aspects of molecular genetics technologies.
1. How did the discovery of restriction enzymes revolutionize DNA technology, and why are “sticky ends” particularly valuable for researchers?
Answer:
- Discovery and Revolution: Restriction enzymes (also called restriction endonucleases) were first discovered in bacteria, where they serve as a defense mechanism against viral DNA. Their ability to cut DNA at specific nucleotide sequences changed molecular biology by allowing scientists to excise and rejoin DNA fragments in a controlled, predictable way.
- Sticky Ends: Some restriction enzymes create overhanging single-stranded ends known as “sticky ends.” These ends have unpaired bases that can form hydrogen bonds with complementary sequences cut by the same enzyme. This specificity simplifies the process of joining different DNA fragments (ligation), greatly facilitating the construction of recombinant DNA molecules. The use of sticky ends therefore streamlines cloning processes and underpins the majority of recombinant DNA techniques, including the creation of genetically modified organisms (GMOs) and recombinant therapeutic proteins.
2. Why is PCR (Polymerase Chain Reaction) described as a cornerstone in modern molecular biology, and what are two major areas where it has had a transformative impact?
Answer:
- Cornerstone in Modern Molecular Biology: PCR allows for the exponential amplification of a specific DNA segment using just a small amount of template. By cycling through denaturation, annealing of primers, and extension by a thermostable DNA polymerase, researchers can generate millions (or even billions) of copies of the target DNA in hours.
- Transformative Impact:
- Medical Diagnosis and Infectious Disease Detection: PCR is used to rapidly identify pathogens (such as viruses and bacteria) in patient samples at very low concentrations, enabling accurate and early disease diagnosis.
- Forensic Science: PCR can amplify trace amounts of DNA from crime scenes (hair, saliva, etc.), making it possible to generate DNA profiles and link evidence to specific individuals, dramatically enhancing the power and reliability of forensic investigations.
3. CRISPR-Cas9 has been hailed as a groundbreaking gene-editing tool. What sets it apart from earlier gene-editing methods, and what potential challenges does its use present?
Answer:
Key Advantages Over Older Methods:
- Guide RNA Specificity: CRISPR uses a short, customizable guide RNA to direct the Cas9 enzyme to virtually any DNA sequence, making it far more versatile than earlier technologies like zinc-finger nucleases or TALENs, which required complex protein engineering.
- Multiplex Editing: Multiple genetic targets can be edited simultaneously in a single cell or organism, accelerating research and potential therapeutic strategies.
- Accessibility and Cost: Designing a guide RNA is relatively straightforward and inexpensive compared to older methods, democratizing gene editing for many labs worldwide.
Potential Challenges:
- Off-Target Effects: Unintentional edits to non-target DNA sites can lead to mutations with unknown consequences.
- Ethical Concerns: Editing human germline cells or releasing genetically modified organisms (e.g., “gene drive” insects) into the wild raises debates about long-term ecological and societal impacts.
- Regulatory Hurdles: Different countries have varying rules; establishing global guidelines is complex, especially for potential germline editing in humans.
4. How do researchers construct a genomic library, and in what scenarios would a genomic library be more advantageous than a cDNA library?
Answer:
Constructing a Genomic Library:
- Extract an organism’s total DNA (the entire genome).
- Cut the DNA with restriction enzymes to create numerous overlapping fragments.
- Insert each fragment into a cloning vector (like a plasmid or a bacteriophage) and introduce these vectors into host cells.
The resulting “library” of host cells each contains a different fragment of the original genome, collectively representing the entire genetic blueprint of the organism.
Advantages over a cDNA Library:
- Non-Coding Regions: A genomic library retains both coding (exons) and non-coding (introns, regulatory sequences) regions, crucial for studying gene regulation, promoter function, or structural genomics.
- Whole Genome Coverage: Ideal for analyzing large-scale genetic organization and identifying unknown genes or regulatory elements. A cDNA library, by contrast, is derived from mRNA and only represents expressed genes.
5. DNA sequencing technologies have evolved rapidly. Compare the Sanger method with next-generation sequencing (NGS) in terms of throughput, cost, and typical use cases.
Answer:
Sanger Sequencing (Dideoxy Method):
- Throughput and Cost: Typically handles shorter reads (up to about 1,000 bases) and is more expensive per base.
- Use Cases: Ideal for smaller projects requiring high accuracy, such as verifying cloned genes or validating specific PCR-amplified segments.
- Method Basics: Relies on chain-terminating dideoxynucleotides labeled with dyes for each base. Results are read via capillary electrophoresis.
Next-Generation Sequencing (NGS):
- Throughput and Cost: Can generate billions of short reads (50–300 bases each) in parallel, drastically reducing the cost per base and increasing data output.
- Use Cases: Suitable for large-scale genomics projects (e.g., whole-genome sequencing, transcriptome analysis, metagenomics), which require sequencing of many genes or entire genomes simultaneously.
- Method Basics: Various platforms exist (Illumina, Ion Torrent, etc.), but all feature massively parallel sequencing of fragmented DNA with sophisticated software to assemble reads.
6. Gene therapy holds enormous promise, but what obstacles must be overcome to make it a routine treatment for genetic diseases?
Answer:
- Delivery Mechanisms: Safely delivering new genes into a patient’s cells remains a challenge. Viral vectors (e.g., adeno-associated virus) are efficient but can trigger immune responses. Non-viral methods (e.g., liposomes, nanoparticles) often struggle with low delivery efficiency.
- Target Specificity and Control: Even after successful delivery, ensuring the therapeutic gene is expressed in the right tissues at the right level is complex. Uncontrolled expression can lead to unwanted effects or toxicity.
- Long-Term Stability: For gene therapy to be curative, the introduced genetic changes must persist over the patient’s lifetime, especially for disorders that affect dividing cells.
- Ethical and Regulatory Hurdles: Germline modifications and potential long-term side effects demand careful oversight and large-scale clinical trials to validate both efficacy and safety.
7. How does DNA fingerprinting (a.k.a. DNA profiling) work, and why is it considered highly reliable in forensics?
Answer:
- Mechanism:
- Extraction and Amplification: DNA is extracted from biological materials (blood, hair, skin cells), then regions of interest—particularly short tandem repeats (STRs)—are amplified via PCR.
- Separation and Detection: The amplified STR fragments are separated by size using capillary electrophoresis. Each individual’s combination of STR sizes across multiple loci forms a unique “profile.”
- Reliability:
- High Polymorphism: STR regions vary greatly among individuals, minimizing the probability of two unrelated people having the exact same profile.
- Statistical Validation: Forensic labs typically examine a dozen or more STR sites, making the chance of accidental match extremely low.
- Standardization: Globally recognized protocols (like CODIS in the U.S.) ensure consistency and scientific rigor.
8. What are genetically modified organisms (GMOs), and what are two key arguments for and against their use in agriculture?
Answer:
Definition: GMOs are organisms (plants, animals, or microbes) whose genomes have been altered using recombinant DNA technology, gene editing (e.g., CRISPR), or other molecular methods to exhibit desired traits (e.g., pest resistance, enhanced nutrition).
Arguments For:
- Improved Crop Yields: Transgenic crops can resist pests or harsh conditions, reducing losses and potentially aiding food security in regions with limited resources.
- Lower Chemical Usage: Pest-resistant plants can diminish the need for pesticides, which can lead to environmental benefits and lower production costs.
Arguments Against:
- Ecological Concerns: There is a risk of transgenes spreading to wild species or off-target organisms, potentially disrupting ecosystems.
- Long-Term Health Effects: Although scientific consensus suggests GMOs are safe to eat, some remain concerned about possible unknown health impacts or allergic reactions.
9. Why is cloning an organism via somatic cell nuclear transfer (SCNT) challenging, and how has it contributed to scientific research?
Answer:
Challenges of SCNT:
- Technical Complexity: The delicate process requires enucleating an egg cell, introducing a somatic cell nucleus, and chemically or electrically stimulating it to begin embryonic development.
- Low Success Rates: Many attempts fail during embryogenesis. Even successful clones can face developmental abnormalities.
- Ethical Considerations: Cloning research in higher mammals, especially primates, raises concerns about animal welfare and human reproductive cloning.
Scientific Contributions:
- Basic Biology: Allows the study of nuclear reprogramming and developmental processes, offering insights into how genes are switched on or off during embryogenesis.
- Biomedical Models: Cloned animals (e.g., Dolly the Sheep) have provided valuable disease models for testing new drugs and therapeutic interventions.
10. In DNA microarray (gene chip) analysis, how does comparing gene expression profiles across conditions help in understanding diseases and designing treatments?
Answer:
Mechanism of DNA Microarrays:
- Probe Arrays: Thousands of known gene sequences are immobilized on a solid surface.
- Hybridization: Fluorescently labeled cDNA samples from different cells (e.g., diseased vs. healthy) bind to complementary DNA probes on the array.
- Analysis: The fluorescence patterns indicate which genes are over- or under-expressed under each condition.
Value in Disease Study and Treatment Design:
- Biomarker Identification: Identifying gene expression changes helps pinpoint potential disease markers for early diagnosis.
- Target Discovery: Genes significantly upregulated in disease states may encode proteins that can be targeted by new drugs.
- Personalized Medicine: Microarray data can guide more precise treatments based on an individual’s unique expression profile.
11. What is “gene drive” technology, and how could it be both a powerful and potentially risky tool for controlling populations of disease vectors?
Answer:
Definition of Gene Drive: A gene drive biases the inheritance of a particular trait so that it spreads rapidly through a population, overcoming the usual 50% inheritance probability during sexual reproduction. CRISPR-based gene drives can insert specific genes into nearly all offspring, ensuring the trait’s dominance.
Powerful Tool:
- Disease Control: In mosquitoes transmitting malaria or dengue, gene drives could spread sterility or resistance genes, drastically reducing vector populations or transmission rates.
- Ecological Management: Could be used to control invasive species or pests that harm agriculture.
Potential Risks:
- Unintended Ecological Consequences: Altering or eliminating a species could disrupt ecosystems in unforeseen ways.
- Loss of Control: Once introduced, a gene drive might cross borders or mutate, becoming hard to contain.
- Ethical Dilemmas: Deciding whether and how to deploy such a powerful tool requires global consensus and responsible governance.
12. How does the rapid pace of innovation in DNA technology challenge existing regulatory frameworks, and what might be needed to ensure responsible use?
Answer:
Regulatory Challenges:
- Technology Outpacing Legislation: Laws and guidelines often lag behind new breakthroughs, leaving grey areas for emerging techniques like CRISPR-based gene editing.
- Complex Ethical Landscape: Human germline editing, GM crops, and synthetic biology involve moral questions about altering nature and future generations. Current regulations differ significantly among countries, complicating international cooperation.
Ensuring Responsible Use:
- Adaptive Policies: Regulators need flexible, science-based guidelines that can be updated quickly as new discoveries arise.
- Public Engagement and Transparency: Inclusion of diverse stakeholders—scientists, policymakers, ethicists, and the public—fosters informed decision-making and public trust.
- Global Collaboration: International treaties or agreements may be necessary for issues like gene drives or germline editing, which can have cross-border impacts and ramifications for human health and biodiversity.
These 12 questions and answers delve into the intricacies, benefits, and ethical dimensions of DNA technology, encouraging nuanced discussions about both the scientific principles involved and the broader implications for society.